What is the Formulation of Lipid Nanoparticles (LNPs)?
In recent years, Lipid nanoparticles (LNPs) have been successfully used as delivery platforms for RNA vaccines and therapeutics. Naked RNA is a negatively charged hydrophilic macromolecule. Due to the electrostatic repulsion of the cell membrane, it is difficult to enter cells and is easily degraded rapidly by ubiquitous RNases. Therefore, a protective shell is required to gain entry into the cell. Because the cell membrane is mainly composed of lipids, using lipid vesicles to encapsulate RNA can pass through the cell membrane and release the RNA into the cytoplasm. Therefore, the vesicle should first be a positively charged lipid capable of binding negatively charged RNA. However, vesicles composed of permanently cationic lipids can cause cytotoxicity due to electrostatic interactions with negatively charged cell membranes, and lipid structures have evolved to become positively charged molecules in response to the acidic environment of the endosome.
LNP Ingredients and Formulations
Currently, the lipid components of classic LNPs usually include four types: ionizable lipids (or cationic lipids), auxiliary lipids, cholesterol, and pegylated lipids (PEG-lipids).
Cationic Lipids (CLs) and Ionizable Lipids (ILs)
Cationic lipids (CLs) and ionizable lipids (ILs) can initiate the first step of self-assembly through electrostatic interactions. Lipoplexes containing cationic lipids are still widely used for nucleic acid delivery. However, due to toxicity issues and lack of efficacy in vivo, they have been largely replaced by pH-responsive ionizable lipids. In LNP formulations, ionizable lipids behave neutrally at physiological pH and are positively charged in the acidic environment of endosomes. The pH-dependent ionization ability makes ionizable lipids suitable materials for nucleic acid delivery due to largely improved effectiveness and toxicity profiles. The proportion of ionizable lipids in the formulation is usually 30%-50% of the total lipids. Currently, much research is devoted to fine-tuning the properties of ionizable lipids to further improve efficiency, especially in improving delivery efficiency in hard-to-reach human tissues. The overall structure of cationic lipids and ionizable lipids can be divided into three parts: (1) head, (2) connecting fragment, and (3) tail.
- Head
The head groups of ionizable lipids usually carry a positive charge. The size and charge density of the head group are mainly involved in the process of encapsulating nucleic acids, stabilizing LNP, interacting with cell membranes, and promoting endosomal escape. Common ionizable lipids contain only one head group and sometimes several head groups. Typical head groups include amines (primary, secondary, tertiary, quaternary), guanidine, heterocyclic groups, etc. The ionizable lipids used clinically (DLin-MC3-DMA, SM-102, ALC-0315) contain a tertiary amine head group and can undergo pH-dependent ionization. The head group of ALC-0315 and SM-102 also contains a terminal hydroxyl group, which can reduce the hydration of the head group and improve hydrogen bonding interactions with nucleic acids, thereby potentially improving transfection ability.
- Connect Fragments
Connecting segments can connect the head to the tail, and are sometimes hidden within the tail (SM-102, ALC-0315). The linker fragment will affect the stability, biodegradability, cytotoxicity and transfection efficiency of LNP. Ionizable lipids may contain one or more linker segments; most ionizable lipids contain only one type of linker segment, probably due to ease of synthesis. Linking fragments can be divided into two categories: non-biodegradable (such as ethers and carbamates) and biodegradable (such as esters, amides and thiols). Biodegradable linker fragments are preferred because they are generally cleared rapidly from the body, allow for multiple doses, and reduce side effects. DLin-MC3-DMA, ALC-0315 and SM-102 all contain ester linkage fragments. Among them, modifications around the ester group of SM-102 will affect the clearance of LNP, formulation stability and transfection efficiency.
- Tail
The hydrophobic tail affects pKa, lipophilicity, mobility, and fusogenicity, thereby affecting LNP formation and potency. Ionizable lipids usually contain 1 to 4 hydrophobic tails containing 8 to 20 carbon atoms. The tail can be a saturated/or unsaturated fatty chain, and the degree of unsaturation can affect nucleic acid delivery by modulating properties associated with membrane destabilization. DLin-MC3-DMA has two linoleyl tails, while ALC0315 and SM-102 have two branched saturated tails, which are thought to have a tapered geometry that promotes endosomal membrane instability and intracytoplasmic release of nucleic acids.
Cholesterol
Cholesterol is a naturally abundant component of cell membranes and is often used as a structural lipid in LNP formulations. Cholesterol accounts for about 20-50% in LNP formula. Cholesterol is a rich component of animal cell membranes and is usually extracted in large quantities from natural raw materials such as wool. The role of cholesterol in cellular uptake has been overlooked for a long time. The study found that replacing cholesterol in LNP formulations with natural phytosterols, such as beta-sitosterol and oxidized cholesterol derivatives, significantly improved mRNA delivery, possibly due to different lipid trafficking in the endocytic pathway and LNP endosomal escape. Enhanced results. Although it is not entirely clear why β-sitosterol-containing LNPs are more likely to escape the lysosomal pathway, their shape polymorphism and multilayered structural organization may deform the endosomal membrane or prolong the time frame of nucleic acid release. Cholesterol is mainly present in the outer shell of LNPs, which explains why modifications to the sterol structure may cause tissue changes on the LNP surface.
- Our Cholesterol Products
Catalog | Product Name | CAS Number | Category |
---|---|---|---|
BPG-3283 | 22-alpha-Hydroxy Cholesterol | 17954-98-2 | Glycosylated Sterols |
BPG-3296 | 24-Methylenecholesterol | 474-63-5 | Oxysterols |
BPG-3282 | 7α-Hydroxy Cholesterol | 566-26-7 | Cholesteryl Esters |
BPG-3284 | 7β-Hydroxy Cholesterol | 566-27-8 | Glycosylated Sterols |
BPG-3288 | 7-Ketocholesterol | 566-28-9 | Natural Sterols |
BPG-3290 | 24(S)-Hydroxycholesterol | 474-73-7 | Natural Sterols |
BPG-3303 | 7-keto-27-hydroxycholesterol | 240129-30-0 | Oxysterols |
BPG-3311 | 7α,24(S)-dihydroxycholesterol | 245523-67-5 | Oxysterols |
BPG-3302 | 7α,27-dihydroxycholesterol | 4725-24-0 | Oxysterols |
BPG-3301 | Galactosyl Cholesterol | 51704-23-5 | Oxysterols |
Phospholipids
Phospholipids help encapsulate nucleic acids and stabilize LNPs. Compared with other components, there are relatively few studies on them, and they usually account for 10%-20% of the total lipids in the formula. Phospholipids are also commonly used as structural lipids in LNP formulations because they can spontaneously organize into lipid bilayers and their higher phase transition temperatures enhance the membrane stability of LNPs. Similar to cell membranes, phospholipids are located at the periphery of LNPs. These lipids are usually semi-synthetic. For example, phosphatidylcholine is often derived from natural sources such as egg yolks and soybeans, and can be chemically modified (such as adding a fatty acid tail).
- Our Phospholipid Products
Catalog | Product Name | CAS Number | Molecular Formula |
---|---|---|---|
BPG-3253 | 1-O-Hexadecyl-sn-glycero-3-phosphocholine | 52691-62-0 | C24H52NO6P |
BPG-3271 | 3-hexanoyl-NBD Cholesterol | 201731-19-3 | C39H58N4O5 |
BPG-3270 | 3-dodecanoyl-NBD Cholesterol | 1246303-05-8 | C45H70N4O5 |
BPG-3272 | 16:0 TopFluor® cholesterol | 2260795-57-9 | C52H81BF2N2O2 |
BPG-3273 | 18:2 TopFluor® cholesterol | 2260795-60-4 | C54H81BF2N2O2 |
BPG-3269 | 25-(C4 TopFluor®) 25-OH cholesterol | 2260795-67-1 | C44H65BF2N2O3 |
BPG-3274 | TopFluor® TMR cholesterol | 2342615-73-8 | C43H56BF2N3O3 |
1,2-Distearoyl-sn-glycero-3-phosphocholine (DSPC) is a structural lipid in LNP clinically used in the siRNA therapy Onpattro and the SARS-CoV-2 mRNA vaccine. The structure of DSPC consists of a phosphatidylcholine head group and two saturated 18-carbon tails that form a tightly packed lipid bilayer. In LNP, DSPC is mainly located on the surface of the nanoparticles and at the edge of the core of the nanoparticles. 1,2 Dioleoyl-sn-glycero-3-phosphoethanolamine (DOPE) is another phospholipid commonly used in preclinical studies of LNP. The unsaturated tail of DOPE not only forms a more fluid lipid layer, but also can form a hexagonal phase (HII) organizational form. The HII phase can promote the fusion of the lipid membrane and the endosomal membrane, leading to the cytoplasmic release of nucleic acids.
PEG-Lipid
PEG-modified lipids (PEG-lipids) are an important component of LNPs that regulate half-life and cellular uptake. When LNPs are assembled, the PEG chains are located on the shell of the nanoparticles due to their hydrophilicity and large size. Like other nanocarriers, PEG provides an external polymeric layer to LNPs to prevent serum protein adsorption and uptake by the mononuclear phagocyte system, prolonging circulation time in the body. PEG also prevents the aggregation of nanoparticles during storage and in the blood. Furthermore, the amount of PEG-lipid may determine the particle size. Another potential purpose of PEG-lipids is to achieve surface functionalization of LNPs. Functionalized PEG-lipids can bioconjugate LNPs with ligands or biomacromolecules. For example, Singh et al. used DSPE-PEG-amine to couple with hyaluronic acid to achieve tumor targeting. Parhiz et al. used DSPE-PEG-maleimide to couple with antibodies. Although PEG is important for the stability and bioconjugation of LNPs, its desorption is also critical for cell transfection. PEG shed from LNPs can be opsonized by serum proteins such as apolipoproteins and albumin, which are key effectors of receptor-mediated endocytosis of LNPs. Akinc et al. confirmed that ApoE binds to LNP to achieve low-density lipoprotein receptor (LDLR)-mediated internalization in hepatocytes.
Since PEG-lipid can inhibit the binding of ApoE to LNP, excess PEG-lipid may have adverse effects on the cellular uptake and transfection of LNP. LNPs containing less PEG-lipid appear to deliver nucleic acids more efficiently due to easy binding to ApoE. The lipid chain length of PEG-lipids is also an important factor in determining the desorption rate. Mui et al. reported that the degree of PEG shedding from the LNP membrane is inversely proportional to the length of the lipid chain in the PEG-lipid, because the hydrophobic interaction between the PEG-lipid and the LNP membrane increases with the length of the lipid chain of the PEG-lipid. Increase by increase.
One of BOC Sciences' key strengths is the wide range of high-quality lipids available, and our product catalog includes phospholipids, glycolipids, sphingolipids and neutral lipids to meet the specific needs of researchers. In addition to lipid products, BOC Sciences offers a range of lipid services to support researchers in their work. These services include lipid synthesis, lipid extraction, lipid analysis, and lipidomics. If you are interested in our lipid products or lipid services, please contact us for more information.
Why BOC Sciences?
-
Large Stock
More than 2000+ products in inventory
-
Global Delivery
Warehouses in multiple cities to ensure fast delivery
-
mg to kg
Multi specification for academic research and industrial production
-
24/7 Technical Support
Strict process parameter control to ensure product quality
Technical Support
- Aqueous Two-Phase System (ATPS) Technique
- Capillary Electrophoresis (CE) Technique
- Enzyme-linked immunosorbent assay (ELISA) Technique
- High performance liquid chromatography (HPLC) Technique
- Hydrophobic Interaction Chromatography (HIC) Technique
- PEGylated Protein Purification Techniques
- Radiolabeling Technique
- SDS-PAGE Technique
- Ultrafiltration Technique
Products
- Lipids
- PEG Derivatives by Structure
-
PEG Derivatives by Functional Group
- Acrylate/Acrylamide/Methacrylate PEG
- Aldehyde (Ald/CHO)PEG
- Alkyne PEG
- Amino PEG, PEG amine(-NH2)
- Azide PEG, Azido PEG(-N3)
- Biotin PEG
- Boc/Fmoc protected amine PEG
- Carboxylic Acid(-COOH) PEG
- Cholesterol PEG
- DBCO PEG
- DNP PEG
- DSPE PEG
- Epoxide glycidyl ether PEG
- FITC PEG
- Folate PEG
- Halide (chloride, bromide) PEG
- Hydrazide PEG
- Hydroxyl(-OH) PEG
- Maleimide(-MAL) PEG
- NHS ester PEG
- Nitrophenyl carbonate (NPC) PEG
- Norbornene PEG
- Olefin/Alkene/Vinyl PEG
- Orthopyridyl disulfide (OPSS) PEG
- Phosphate PEG
- Rhodamine PEG
- SCM PEG
- Silane PEG
- SPDP PEG
- Sulfonate (tosyl, mesyl, tresyl) PEG
- tert-Butyl protected carboxylate PEG
- Thiol(-SH) PEG
- Vinylsulfone PEG
- PEG Copolymers
-
PEG Raw Materials
- Small-molecule Polyethylene Glycol
- Polyethylene Glycol 1000
- Polyethylene Glycol 10000
- Polyethylene Glycol 1500
- Polyethylene Glycol 200
- Polyethylene Glycol 2000
- Polyethylene Glycol 20000
- Polyethylene Glycol 400
- Polyethylene Glycol 4000
- Polyethylene Glycol 600
- Polyethylene Glycol 6000
- Polyethylene Glycol 800
- Polyethylene Glycol 8000
Resources
-
Technical Information
- Aqueous Two-Phase System (ATPS) Technique
- Capillary Electrophoresis (CE) Technique
- Enzyme-linked immunosorbent assay (ELISA) Technique
- High performance liquid chromatography (HPLC) Technique
- How to Perform Polyethylene Glycol (PEG) Modification?
- Hydrophobic Interaction Chromatography (HIC) Technique
- Introduction of Polyethylene Glycol (PEG)
- Ion Exchange Chromatography (IEX) Technique
- PEG for Chemical Synthesis
- PEG for Cosmetic Application
- PEG for Drug Delivery
- PEG for Imaging Diagnosis
- PEG for Pharmaceutical Preparation
- PEG for Tissue Engineering
- PEG Purification Techniques of Plasmid DNA
- PEGylated Protein Purification Techniques
- Polyethylene Glycol (PEG) Modifier Selection Guide
- Radiolabeling Technique
- SDS-PAGE Technique
- Size Exclusion Chromatography (SEC) Technique
- Ultrafiltration Technique
-
Industry News
- Applications of PEG-DSPE: Drug Carriers and Drug Delivery
- Applications of Polyethylene Glycol (PEG) as Medical Devices
- Cholesterol: Definition, Structure, Synthesis, Types and Functions
- Classification of Lipid-Based Vaccine Adjuvants
- FDA approved PEGylated Products
- FDA-Approved Antibody-Drug Conjugates up to 2024
- How are Liposomes Different from Micelles?
- How Lipid Nanoparticles (LNPs) Deliver RNA Drugs?
- Hyaluronic Acid & PEGylated Hyaluronic Acid
- Ionizable Lipids for RNA Delivery
- Lipid Classification and Drug Delivery Systems
- Lipid Formulations: Key Absorption-Enhancing Technologies in New Drug Development
- Lipid-Drug Conjugates (LDCs) for Nanoparticle Drug Delivery
- Liposome in Drug Delivery
- Overview of Liposome Preparation Process
- PEG in Pharmaceutical Preparations (I): Solvents, Lubricants, Adhesives and More
- PEG in Pharmaceutical Preparations (II): Stabilizers, Plasticizers and Modification Materials
- PEG Linkers in Antibody Drug Conjugates and PROTACs
- PEG-DSPE Block Copolymers and Their Derivatives
- PEGylated Drugs: Definition, Structure, Classification and Benefits
- PEGylated RGD Peptides: A Promising Tool for Targeted Drug Delivery
- Pharmacokinetics and Bioanalysis of PEGylated Drugs
- Polyethylene Glycol (PEG) Modified Targeting Nanomaterials
- Preparation Method of PEG Hydrogel
- The PROTAC Technology in Drug Development
- Vaccines: Definition, History, Ingredients, Types and Mechanism of Action
- What are Lipid Excipients and Their Applications?
- What are Lipid Nanoparticles and Their Applications?
- What are Lipid-Drug Conjugates (LDCs)?
- What are Lipids?
- What are Monodispersed and Polydispersed PEGs?
- What are PEG Lipids?
- What are Phospholipids?
- What are Sterols? - Definition, Structure, Function, Examples and Uses
- What is Biotinylation and Biotinylated PEG?
- What is Click Chemistry?
- What is Hydrogel?
- What is Methoxy Polyethylene Glycol (mPEG)?
- What is Nanogels and Its Applications?
- What is the Formulation of Lipid Nanoparticles (LNPs)?
Our Feature
BOC Sciences supplies a unique variety of PEG derivatives and functional PEG polymers. Our products offer the most diverse collection of reactivity, ready-to-use functionality, and molecular weight options that you will not find anywhere else.
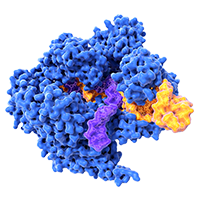
PEGylation of Peptides
and Proteins
Reduce the Immunogenicity of Peptide/Protein Drugs
Learn More
APPLICATIONS
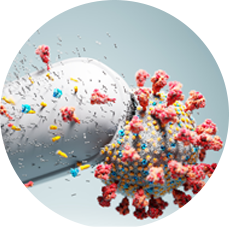
PEG linkers For Drug
Improved Circulation Half-Life
Learn More
Have Customer Reviewed On Us?
Chat With Us