Polyethylene Glycol in Small Molecule-Drug Conjugates
Conjugated drugs are created by combining cytotoxic drugs with targeted ligands, allowing for the targeted delivery of the drug to tumor sites. This approach not only reduces drug toxicity but also enhances therapeutic efficacy, representing a powerful combination of effective killing and precise targeting. Among these, Antibody-Drug Conjugates (ADCs) are the most well-known conjugated drugs, with over a dozen ADC products already approved by the FDA, and hundreds of ADC clinical trials currently underway worldwide. The successful "formula" for antibody-drug conjugates includes a carrier targeting tumors, a substance that kills tumor cells, and a linker connecting the first two components. This concept has inspired the development of additional conjugated drugs, such as Peptide-Drug Conjugates (PDC), composed of homing peptides, cytotoxins, and linkers, as well as Radionuclide-Drug Conjugates (RDC), which combine targeting antibodies or small molecules with radioactive isotopes that can both image and kill via radiation. Currently, another novel approach for the precise targeting delivery of chemotherapy drugs—Small Molecule-Drug Conjugates (SMDC)—is gaining attention in the industry, joining the ranks of popular new conjugated drugs.
Small Molecule-Drug Conjugates
SMDCs typically consist of a small molecule targeting ligand, a cytotoxic agent, and a linker. The key difference between SMDCs and ADCs lies in the targeting ligand; ADCs use large molecule antibodies, while SMDCs use small molecules. The small molecule ligands also determine the distinct pharmacokinetic characteristics of SMDCs, as they are more permeable and can distribute more uniformly throughout tumor tissues, without accumulating in tumors or other normal cells. Any off-target drugs are rapidly expelled from the body, thereby reducing toxicity to normal cells. However, unlike ADCs, selecting the appropriate ligands for SMDCs is challenging; obtaining small molecule ligands is often difficult, primarily relying on derivatives from naturally occurring ligands, such as folate derivatives targeting folate receptors, glutamate derivatives targeting prostate-specific membrane antigen, and somatostatin analogs targeting somatostatin receptors.
Fig. 1. Structure of SMDC (Eur J Med Chem. 2019, 163: 883-895).
Targeting Ligands
The structure of SMDCs includes three components: small molecule targeting ligands, a linker, and cytotoxic molecules (the therapeutic payload). The significant distinction from ADCs lies in the targeting ligand; ADCs utilize large molecule antibodies, whereas SMDCs utilize small molecules. The small molecule ligands also contribute to the unique pharmacokinetic properties of SMDCs, facilitating their ability to permeate and distribute uniformly in tumor tissues, while minimizing accumulation in both tumors and normal cells. The challenge lies in identifying suitable small molecule ligands with high affinity, specificity, appropriate size, and modifiable groups for drug development.
Small Molecule Payloads
Cytotoxic molecules are the core of SMDCs and are crucial for achieving clinical therapeutic value. The cytotoxic agent must exhibit sufficient potency, typically comprising highly toxic compounds that cannot be used as standalone drugs. Furthermore, the cytotoxic agent should possess adequate water solubility while maintaining relative stability in circulation. Additionally, it should have modifiable sites for functional groups to connect with targeting ligands. The requirements for this part of SMDCs are similar to those for ADCs, thus, compounds like vincristine, paclitaxel, mitomycin C, epirubicin, microtubule inhibitors, and the SN-38 metabolite of irinotecan are often utilized.
Linkers
The linker in SMDCs serves as the connecting arm between the targeting ligand and the therapeutic payload, typically comprising a spacer and a cleavable moiety. To maintain the activity of the targeting ligand and the efficacy of the payload, the linker must be meticulously designed to optimize drug release, pharmacokinetics, and pharmacodynamics. The spacer usually acts as a connector between the targeting ligand and the cleavable arm and is crucial for maintaining receptor binding in SMDCs. An inappropriate spacer can lead to reduced binding affinity due to unnecessary intramolecular associations. Moreover, the spacer can enhance the hydrophilicity of SMDCs; since targeting ligands and therapeutic payloads are typically hydrophobic to maximize membrane permeability and receptor affinity, incorporating hydrophilic spacers like polysaccharides, hydrophilic amino acids, polyethylene glycol (PEG), and peptidoglycan can improve the hydrophilic potential of SMDCs. Additionally, the design of the spacer helps to reduce the cytotoxicity of the payload; without receptor-mediated endocytosis, polar conjugates struggle to penetrate cell membranes. However, the characteristics of the spacer remain unclear; in some cases, rigid structures may outperform flexible ones. The effectiveness of SMDCs relies on the ability of the cleavable arm to release the cytotoxic drug from the SMDC parent structure at predictable cleavage sites and rates after penetrating target cells. Therefore, similar to ADCs, SMDCs require stability during circulation in vivo. Two types of triggering methods are commonly used in SMDCs: (1) disulfide bond-based linkers, which are among the most successful methods; and (2) designing cleavable moieties sensitive to the pH of target cells, such as acetals that can hydrolyze in the acidic environment found in vivo.
PEG Linkers for SMDCs
BOC Sciences provides a comprehensive selection of PEG linkers specifically designed to enhance the stability and targeting efficiency of SMDCs. Our portfolio of PEG linkers is available in various molecular weights and functionalized forms, enabling precise control over drug release profiles and bioavailability. Leveraging advanced synthesis capabilities, we offer customized PEG linkers optimized for improved solubility, enhanced cell permeability, and minimized immunogenicity. Our commitment to quality ensures that each PEG linker meets stringent industry standards, making BOC Sciences a reliable partner for researchers and developers seeking robust solutions to advance SMDC applications.
Cat. No. | Product Name | CAS No. | Inquiry |
---|---|---|---|
BPG-2095 | Biotin-PEG2-acid | 1365655-89-5 | Inquiry |
BPG-0836 | Azido-PEG9-amine | 1207714-69-9 | Inquiry |
BPG-2108 | m-PEG8-COOH | 1093647-41-6 | Inquiry |
BPG-1886 | Amino-PEG6-alcohol | 39160-70-8 | Inquiry |
BPG-1869 | Propargyl-PEG5-amine | 1589522-46-2 | Inquiry |
BPG-1933 | Mal-PEG1-NHS ester | 1807518-72-4 | Inquiry |
BPG-1718 | Propargyl-PEG2-acid | 1859379-85-3 | Inquiry |
BPG-2224 | Azido-PEG6-NHS ester | 2055014-64-5 | Inquiry |
BPG-2118 | Azide-PEG5-Tos | 236754-49-7 | Inquiry |
BPG-1851 | Azido-PEG5-alcohol | 86770-68-5 | Inquiry |
Advantages and Disadvantages of SMDCs
SMDCs have certain limitations that have yet to be overcome, particularly the difficulty in obtaining suitable receptors and ligands, which restricts the speed of SMDC drug development due to the scarcity of targets and indications. However, the structural composition and mechanisms of action of SMDCs are very similar to those of ADCs, and they also have unique advantages as small molecule drugs:
- Cost Advantage: The small molecule composition of SMDCs makes them easier to synthesize compared to antibody drugs. Their industrial production technologies are simpler, making it easier to control production costs.
- Safety Advantage: SMDCs avoid the immunogenicity issues associated with biologics, leading to improved safety profiles.
- Enhanced Permeability: With a lower molecular weight, SMDCs exhibit better cell permeability, allowing them to penetrate tumor tissues more easily through passive diffusion and achieve more uniform distribution.
- Stability In Vivo and In Vitro: While large molecules tend to accumulate in metabolic tissues, small molecules generally do not accumulate within cells or tissues, leading to simpler and faster metabolic pathways. Even when a small fraction of the drug is off-target, it is quickly expelled from the body, resulting in lower toxicity to normal cells.
- Precise Payload (Cytotoxic Agents): The effective payload of each ADC molecule relative to its antibody is often indeterminate, leading to variability in intracellular effective payloads. In contrast, SMDCs usually maintain a precise ratio between the small molecule targeting ligands and their effective payloads.
How Do SMDCs Work?
In terms of mechanism, SMDCs and ADCs are highly consistent. For example, in SMDCs targeting folate receptors, these drugs do not enter cells through the reduced folate carrier like normal cells absorb folate. Instead, similar to ADCs, they enter cells via endocytosis by binding to high-affinity folate receptors, followed by the release of cytotoxic molecules upon cleavage, exerting their killing effect, after which the folate receptors recycle back to the cell surface. It is evident that SMDCs share many commonalities with ADCs in terms of molecular composition, mechanisms of action, and related drug requirements. Therefore, during the development of SMDC drugs, referencing the development experiences and methods of ADCs may provide valuable insights.
Fig. 2. Mechanism of action fo SMDC (Eur J Med Chem. 2019, 163: 883-895).
Clinical Trials of SMDCs
The development timeline for SMDC drugs is relatively short, with representative companies including Endocyte, Tarveda, and Bind Therapeutics. Currently, there are nine registered products in the clinical research phase, with three in Phase II trials and five in Phase I trials, primarily developed by small biotech companies like Endocyte and Tarveda, although major pharmaceutical giants such as Merck, Novartis, and BMS are also involved.
Product Name | Company | Targeted Receptor | Cytotoxic Molecule | Clinical Stage | Indications |
---|---|---|---|---|---|
Vintafolide | Merck & Endocyte | Folic acid | Vinblastine | III/IIb | Platinum-resistant ovarian cancer/non-small cell lung cancer |
PEN-866 | Tarveda | Heat shock protein | SN-38 | II | Recurrent and metastatic solid tumors |
177Lu-PSMA-617 | Novartis & Endocyte | Prostate-specific membrane antigen | 177Lu | II | Metastatic castration-resistant prostate cancer |
Epofolate (BMS-753493) | BMS & Endocyte | Folic acid | Epothilone | II | Recurrent and metastatic solid tumors |
EC1456 | Endocyte | Folic acid | Tubulysin | I | Recurrent and metastatic solid tumors |
EC1169 | Endocyte | Prostate-specific membrane antigen | Tubulysin | I | Metastatic castration-resistant prostate cancer |
EC0489 | Endocyte | Folic acid | Vinblastine | I | Recurrent and metastatic solid tumors |
EC0225 | Endocyte | Folic acid | Mitomycin C | I | Recurrent and metastatic solid tumors |
PEN-221 | Tarveda | Somatostatin receptor 2 | Maytansine derivatives DM-1 | I | Digestive tract tumors |
It can be observed that the indications for SMDC product development differ significantly from those of ADCs. While approved ADC drugs are distributed across both solid tumors and hematologic malignancies, SMDC indications are concentrated in solid tumors. Due to their smaller molecular weight, SMDCs may have better distribution and permeability in tumor tissues, which is why solid tumors are chosen as their indications to leverage this advantage. Additionally, the SMDC products entering clinical stages exhibit a higher concentration in the selection of drug binding targets, with the nine products encompassing only four drug binding targets—five targeting folate receptors and two targeting prostate-specific membrane antigens—further confirming the earlier point about the challenges in finding suitable receptors and ligands for SMDCs.
BOC Sciences provides high-quality PEGylation services for drug conjugates, designed to improve their therapeutic stability, solubility, and half-life. Utilizing a broad array of PEG reagents with customizable molecular weights and reactive functional groups, we optimize PEG attachment to enhance the bioavailability and targeting precision of drug conjugates. Our advanced PEGylation techniques ensure minimized immunogenicity and optimized pharmacokinetics, contributing to safer and more effective drug delivery systems. With robust technical support and rigorous quality control, BOC Sciences offers scalable and tailored PEGylation solutions that meet diverse needs in drug conjugate development.
** Recommended Products **
Cat. No. | Product Name | CAS No. | Inquiry |
---|---|---|---|
BPG-0684 | Amino-PEG12-amine | 361543-12-6 | Inquiry |
BPG-1738 | Amino-PEG3-amine | 929-75-9 | Inquiry |
BPG-1811 | Amino-PEG4-amine | 68960-97-4 | Inquiry |
BPG-0685 | Amino-PEG11-COOH | 1616426-12-0 | Inquiry |
BPG-1703 | Amino-PEG1-acid | 144942-89-2 | Inquiry |
BPG-1722 | Amino-PEG2-propionic acid | 791028-27-8 | Inquiry |
BPG-0854 | Azido-PEG10-Acid | 1644163-57-4 | Inquiry |
BPG-0855 | Azido-PEG12-Acid | 2152679-71-3 | Inquiry |
BPG-1710 | Azido-PEG1-acid | 1393330-34-1 | Inquiry |
BPG-1719 | Azido-PEG2-amine | 166388-57-4 | Inquiry |
BPG-1767 | Azido-PEG3-amine | 134179-38-7 | Inquiry |
BPG-1849 | Azido-PEG4-amine | 951671-92-4 | Inquiry |
BPG-1401 | Bromo-PEG11-alcohol | 1556847-51-8 | Inquiry |
BPG-1403 | Bromo-PEG8-alcohol | 1527515-88-3 | Inquiry |
BPG-1717 | Bromo-PEG2-alcohol | 57641-66-4 | Inquiry |
BPG-1762 | Bromo-PEG3-alcohol | 57641-67-5 | Inquiry |
BPG-0370 | Mal-PEG3-NHS ester | 1537892-36-6 | Inquiry |
BPG-1933 | Mal-PEG1-NHS ester | 1807518-72-4 | Inquiry |
BPG-3586 | Mal-PEG6-NHS ester | 1599472-25-9 | Inquiry |
BPG-3588 | Mal-PEG4-NHS | 1325208-25-0 | Inquiry |
Reference
- Zhuang, C. et al. Small molecule-drug conjugates: A novel strategy for cancer-targeted treatment. Eur J Med Chem. 2019, 163: 883-895.
Why BOC Sciences?
-
Large Stock
More than 2000+ products in inventory
-
Global Delivery
Warehouses in multiple cities to ensure fast delivery
-
mg to kg
Multi specification for academic research and industrial production
-
24/7 Technical Support
Strict process parameter control to ensure product quality
Technical Support
- Aqueous Two-Phase System (ATPS) Technique
- Capillary Electrophoresis (CE) Technique
- Enzyme-linked immunosorbent assay (ELISA) Technique
- High performance liquid chromatography (HPLC) Technique
- Hydrophobic Interaction Chromatography (HIC) Technique
- PEGylated Protein Purification Techniques
- Radiolabeling Technique
- SDS-PAGE Technique
- Ultrafiltration Technique
Products
- Lipids
- PEG Derivatives by Structure
-
PEG Derivatives by Functional Group
- Acrylate/Acrylamide/Methacrylate PEG
- Aldehyde (Ald/CHO)PEG
- Alkyne PEG
- Amino PEG, PEG amine(-NH2)
- Azide PEG, Azido PEG(-N3)
- Biotin PEG
- Boc/Fmoc protected amine PEG
- Carboxylic Acid(-COOH) PEG
- Cholesterol PEG
- DBCO PEG
- DNP PEG
- DSPE PEG
- Epoxide glycidyl ether PEG
- FITC PEG
- Folate PEG
- Halide (chloride, bromide) PEG
- Hydrazide PEG
- Hydroxyl(-OH) PEG
- Maleimide(-MAL) PEG
- NHS ester PEG
- Nitrophenyl carbonate (NPC) PEG
- Norbornene PEG
- Olefin/Alkene/Vinyl PEG
- Orthopyridyl disulfide (OPSS) PEG
- Phosphate PEG
- Rhodamine PEG
- SCM PEG
- Silane PEG
- SPDP PEG
- Sulfonate (tosyl, mesyl, tresyl) PEG
- tert-Butyl protected carboxylate PEG
- Thiol(-SH) PEG
- Vinylsulfone PEG
- PEG Copolymers
-
PEG Raw Materials
- Small-molecule Polyethylene Glycol
- Polyethylene Glycol 1000
- Polyethylene Glycol 10000
- Polyethylene Glycol 1500
- Polyethylene Glycol 200
- Polyethylene Glycol 2000
- Polyethylene Glycol 20000
- Polyethylene Glycol 400
- Polyethylene Glycol 4000
- Polyethylene Glycol 600
- Polyethylene Glycol 6000
- Polyethylene Glycol 800
- Polyethylene Glycol 8000
Resources
-
Technical Information
- Aqueous Two-Phase System (ATPS) Technique
- Capillary Electrophoresis (CE) Technique
- Enzyme-linked immunosorbent assay (ELISA) Technique
- High performance liquid chromatography (HPLC) Technique
- How to Perform Polyethylene Glycol (PEG) Modification?
- Hydrophobic Interaction Chromatography (HIC) Technique
- Introduction of Polyethylene Glycol (PEG)
- Ion Exchange Chromatography (IEX) Technique
- PEG for Chemical Synthesis
- PEG for Cosmetic Application
- PEG for Drug Delivery
- PEG for Imaging Diagnosis
- PEG for Pharmaceutical Preparation
- PEG for Tissue Engineering
- PEG Purification Techniques of Plasmid DNA
- PEGylated Protein Purification Techniques
- Polyethylene Glycol (PEG) Modifier Selection Guide
- Radiolabeling Technique
- SDS-PAGE Technique
- Size Exclusion Chromatography (SEC) Technique
- Ultrafiltration Technique
-
Industry News
- Applications of PEG-DSPE: Drug Carriers and Drug Delivery
- Applications of Polyethylene Glycol (PEG) as Medical Devices
- Cholesterol: Definition, Structure, Synthesis, Types and Functions
- Classification of Lipid-Based Vaccine Adjuvants
- FDA approved PEGylated Products
- FDA-Approved Antibody-Drug Conjugates up to 2024
- How are Liposomes Different from Micelles?
- How Lipid Nanoparticles (LNPs) Deliver RNA Drugs?
- Hyaluronic Acid & PEGylated Hyaluronic Acid
- Ionizable Lipids for RNA Delivery
- Lipid Classification and Drug Delivery Systems
- Lipid Formulations: Key Absorption-Enhancing Technologies in New Drug Development
- Lipid-Drug Conjugates (LDCs) for Nanoparticle Drug Delivery
- Liposome in Drug Delivery
- Overview of Liposome Preparation Process
- PEG in Pharmaceutical Preparations (I): Solvents, Lubricants, Adhesives and More
- PEG in Pharmaceutical Preparations (II): Stabilizers, Plasticizers and Modification Materials
- PEG Linkers in Antibody Drug Conjugates and PROTACs
- PEG-DSPE Block Copolymers and Their Derivatives
- PEGylated Drugs: Definition, Structure, Classification and Benefits
- PEGylated RGD Peptides: A Promising Tool for Targeted Drug Delivery
- Pharmacokinetics and Bioanalysis of PEGylated Drugs
- Polyethylene Glycol (PEG) Modified Targeting Nanomaterials
- Preparation Method of PEG Hydrogel
- The PROTAC Technology in Drug Development
- Vaccines: Definition, History, Ingredients, Types and Mechanism of Action
- What are Lipid Excipients and Their Applications?
- What are Lipid Nanoparticles and Their Applications?
- What are Lipid-Drug Conjugates (LDCs)?
- What are Lipids?
- What are Monodispersed and Polydispersed PEGs?
- What are PEG Lipids?
- What are Phospholipids?
- What are Sterols? - Definition, Structure, Function, Examples and Uses
- What is Biotinylation and Biotinylated PEG?
- What is Click Chemistry?
- What is Hydrogel?
- What is Methoxy Polyethylene Glycol (mPEG)?
- What is Nanogels and Its Applications?
- What is the Formulation of Lipid Nanoparticles (LNPs)?
Our Feature
BOC Sciences supplies a unique variety of PEG derivatives and functional PEG polymers. Our products offer the most diverse collection of reactivity, ready-to-use functionality, and molecular weight options that you will not find anywhere else.
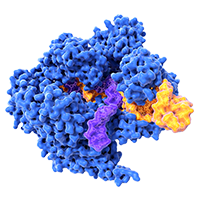
PEGylation of Peptides
and Proteins
Reduce the Immunogenicity of Peptide/Protein Drugs
Learn More
APPLICATIONS
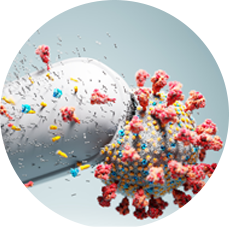
PEG linkers For Drug
Improved Circulation Half-Life
Learn More
Have Customer Reviewed On Us?
Chat With Us