Polyethylene Glycol in 3D Bioprinting
3D bioprinting is the ultimate biomanufacturing technology in the field of tissue engineering and regeneration, using intelligent bioinks and bioprinting systems to construct tissues or organs. This technology has a wide range of biomedical applications, including orthopedics, cardiovascular, skin, cartilage, dentistry and various soft tissue engineering fields, including but not limited to pancreatic and liver tissue engineering applications.
What is 3D Bioprinting?
3D bioprinting is an innovative additive manufacturing technology that combines the precision manufacturing capabilities of 3D printing with cutting-edge biological sciences. It aims to construct a three-dimensional structure with biological functions by layer-by-layer construction. Unlike traditional 3D printing, which mainly relies on materials such as plastics and metals, the core of 3D bioprinting is the use of bioinks, which usually contain living cells, biomaterials (such as hydrogels) and bioactive factors that promote cell growth. By combining these biomaterials with computer-designed models, 3D bioprinting can accurately reconstruct the complex structure and function of human tissues and even organs. Based on the principle of additive manufacturing, the 3D bioprinting process gradually builds a three-dimensional structure by stacking materials layer by layer. This process involves several key steps:
Fig. 1. 3D bioprinting for regenerative medicine (Regen Biomater. 2024, 11: rbae033).
- Digital Model Creation: 3D data of the target tissue or organ is generated through medical imaging technologies (such as CT or MRI) and modeled precisely using computer-aided design (CAD) software.
- Bio-Ink Preparation: Bio-inks of functional value are chosen for the tissue or organ. The ink has to be mechanically strong and it needs to allow cells to grow and differentiate.
- Printing Process: Using printing (inkjet, extrusion, or photopolymerization) process, bio-ink is transferred layer by layer to a substrate to construct the desired object as per the model.
- Post-Processing and Culturing: Printed tissue is typically infused into a bioreactor where they are heated to the right temperature, nutrients, and growth factors for maturation and functionalization.
3D Bioprinting Advantages
The advantages of 3D bioprinting over conventional manufacturing processes are many. It can do very sophisticated geometric 3D shapes, such as the integration of many different types of cells, multilayer tissue distribution, and microscopic information. Secondly, 3D bioprinting could direct imprint the living cells directly into the printed object, so the printed object was not only formed but also able to mimic living functions. Moreover, since the printing model can be tailored to the patient's own anatomical information, the technology has special promise for personalized medicine.
- Personalized Customization: Since 3D bioprinting works from the patient's own anatomical information, it is possible to create highly individualized tissues and organs. This procedure can lead to a much higher graft matching rate for transplant patients.
- Complex Structure Manufacturing: In contrast to traditional manufacturing, 3D bioprinting can be used to precisely fabricate complex, multilayered, multimaterial and multi-cell type three-dimensional structures, mimicking the heterogeneity and structure of human tissues.
- Shortened R&D Cycle: In drug screening and disease model development, 3D bioprinting can quickly produce in vitro models, thus shortening the drug development cycle and reducing costs.
- Reduction of Animal Testing: By printing human tissue models, the need for animal testing is reduced, optimizing ethical concerns while improving the relevance and accuracy of experimental results.
- Regenerability and Repairability: Using the patient's own cells and biomaterials, tissues and organs printed by 3D bioprinting exhibit excellent regenerative and repair capabilities, thereby reducing the risk of rejection.
3D Bioprinting Methods
The method of 3D bioprinting is the core technology for constructing complex biological structures, and its selection directly affects the precision, efficiency, and biological functionality of the print. Different methods enable the flexible application of various bio-inks through differences in material handling and deposition techniques, meeting the manufacturing needs of different tissues and organs. Currently, the main methods of 3D bioprinting include inkjet printing, extrusion printing, photopolymerization printing, and the latest developments in multi-material and multi-cell printing technologies.
Inkjet Printing
Inkjet printing is a non-contact printing technology that builds three-dimensional structures by ejecting liquid bio-ink in the form of micro-droplets onto a substrate. Inkjet printing uses thermal or piezoelectric technology to form tiny ink droplets. These droplets are precisely positioned in a digitally controlled manner, layer by layer, to form the target structure. Inkjet printing is suitable for low-viscosity bio-inks (such as cell suspensions or hydrogel solutions) and offers high resolution and low cost. This method is widely used for printing vascular networks, skin tissues, and drug screening models. However, the survival rate of cells may be affected by potential thermal shock and shear forces during the inkjet printing process.
Extrusion Printing
Extrusion printing is one of the most commonly used methods in 3D bioprinting due to its wide range of material viscosity adaptability, enabling the handling of various bio-inks. Bio-ink is extruded into a continuous fluid through air pressure, screws, or pistons and deposited onto the target position following a designed path. The advantage of extrusion printing is that it can print high-viscosity materials, such as hydrogels with high cell density or viscous biopolymers. In addition, extrusion printing is suitable for constructing large-size and complex structures, but its resolution is relatively lower. Extrusion printing is commonly used for manufacturing cartilage, bone scaffolds, and multi-layer tissue models, particularly those requiring high mechanical strength for biological scaffolds.
Photopolymerization Printing
Photopolymerization printing is based on the light-polymerizing properties of photosensitive materials. It uses ultraviolet light or lasers to cure bio-inks layer by layer, creating precise three-dimensional structures. This method employs digital light processing (DLP) or stereolithography (SLA) technologies to expose photosensitive hydrogels to specific wavelengths of light, initiating polymerization reactions that form solid biological scaffolds. Photopolymerization printing has very high resolution and is suitable for constructing microstructures and complex microscopic details. However, its material selection is limited, and some photosensitive materials may be toxic to cells. Currently, photopolymerization printing is mainly used for printing vascularized tissues, microfluidic chips, and high-precision organ models.
3D Bioprinting Materials
The core of 3D bio-printing is the selection and development of bio-ink. The properties of bio-ink directly determine the structural integrity, biological functionality and clinical applicability of the printed tissue. Bioink refers to functional materials for 3D bioprinting, including cells, scaffold materials and bioactive factors. The ideal bio-ink should not only have good printability and mechanical properties, but also support cell survival, proliferation and differentiation, as well as biocompatibility and appropriate degradation characteristics. According to their origin and properties, 3D bioprinting materials can be divided into natural polymers, synthetic polymers, cell materials and composite materials.
Natural Polymers
Natural polymers are derived from biological sources and have good biocompatibility, mimicking the extracellular matrix (ECM) microenvironment to support cell growth. Common natural polymers include:
Collagen | Collagen, the major ingredient of the extracellular matrix, is commonly used for soft tissue construction. It's beneficial because it's highly biocompatible and biodegradable and allows the cell to adhere and multiply. But collagen is mechanically poor and normally needs to be mixed with other materials for support. |
Gelatin | Gelatin is an collagen derivative, thermoreversible so it can change its gel states by heating it. It is widely used in cartilage and skin tissue printing, but because of its high water solubility, it must be chemically adapted for stability. |
Fibrin | Fibrin has excellent hemostatic and wound-healing properties, making it suitable for vascular tissue printing, particularly in cardiovascular and skin repair applications. |
Alginate | Alginate is a natural polymer that forms gels via ionic crosslinking. It is commonly used in soft tissue printing, offering good biocompatibility and mechanical stability, but it often needs to be combined with other materials to improve cell adhesion. |
Synthetic Polymers
Synthetic polymers are highly regarded for their controllable chemical structures and mechanical properties. These materials offer great design flexibility, allowing adjustment of degradation rates and mechanical strength based on requirements.
Polyethylene Glycol (PEG) | PEG is a water-soluble polymer with excellent biocompatibility and anti-protein adsorption properties. Through chemical modification, PEG can form hydrogels, used for printing complex three-dimensional structures that support cell survival and tissue formation. |
Poly(lactic-co-glycolic acid) (PLGA) | PLGA is a biodegradable material commonly used for printing hard tissues such as bones or teeth. Its degradation products are harmless to the body, and the degradation rate can be controlled by adjusting the molecular weight and copolymer ratio. However, PLGA lacks cell adhesion sites and typically needs to be combined with other materials to improve cell compatibility. |
Polyurethane (PU) | PU is an elastic material with good mechanical properties and biocompatibility, suitable for printing cartilage and vascular tissues. |
Cellular Materials
Cells are one of the most crucial components in 3D bioprinting, providing life and functionality to the printed structures. Common cellular materials include:
Stem Cells | Stem cells have self-renewal and multi-directional differentiation potential, making them essential for 3D bioprinting. Embryonic stem cells and induced pluripotent stem cells (iPSCs), due to their robust differentiation capabilities, are widely used to construct various tissues, such as neural, cardiac, and liver tissues. |
Primary Cells | Primary cells are directly sourced from patient tissues, offering better simulation of the in vivo environment. These cells are suitable for personalized medicine and disease model construction. For example, grafts printed using a patient's skin cells can significantly reduce immune rejection. |
Co-cultured Cell Lines | To simulate the tissue microenvironment, multiple cell types (e.g., fibroblasts and endothelial cells) are often co-cultured and printed together to create functional multi-cellular tissue constructs. |
Composite Materials
Composite materials combine natural and synthetic polymers, combining the advantages of both, and are currently a hot research area. These materials not only have good biocompatibility and cell support capabilities but also exhibit tunable mechanical properties and degradation rates.
Alginate-Gelatin Composite | This composite combines the mechanical stability of alginate with the cell adhesion properties of gelatin, making it suitable for printing cartilage or skin tissues. |
Fibrin-PEG Composite | Combining the biological activity of fibrin with the structural controllability of PEG, this composite is ideal for vascularized and complex three-dimensional structures. |
Ceramic-Polymer Composite | In bone tissue engineering, inorganic materials like hydroxyapatite are often combined with biopolymers to enhance the mechanical properties and mineralization ability of bone scaffolds. |
Polyethylene Glycol for 3D Bioprinting
Based on the above, PEG and its hydrogels have become a research hotspot in this field due to their excellent physicochemical properties and tunable biological performance. PEG is a synthetic polymer formed by the polymerization of ethylene oxide monomers. Its highly hydrophilic nature endows hydrogels with an exceptional water absorption capacity, allowing them to absorb up to a thousand times their dry weight in water. This characteristic makes PEG hydrogels outstanding in simulating the extracellular matrix (ECM), providing a three-dimensional scaffold that closely mimics the physiological environment for cell growth and metabolism. Additionally, PEG hydrogels can be processed through methods such as photopolymerization, enabling precise shape and mechanical property control in 3D bioprinting. The molecular structure of PEG is highly flexible, and its hydroxyl end groups can be converted into various functional groups, including carboxyl, thiol, and amine groups. This ability for chemical modification gives PEG the potential to interact synergistically with other materials, further enhancing its applications in bioprinting. For example, through photochemical crosslinking, PEG derivatives can form hydrogel networks with excellent mechanical properties and stability, meeting the requirements of biological scaffolds in tissue engineering.
Cat. No. | Product Name | Molecular Weight | Price |
---|---|---|---|
BPG-1402 | PCL-PEG-PCL | PEG MW 400-20k | Inquiry |
BPG-1588 | (γ)PGA-PEG-(γ)PGA | PEG MW 1k-5k | Inquiry |
BPG-1446 | PLA-PEG-PLA | PEG MW 400-20k | Inquiry |
BPG-1481 | PLGA-PEG-PLGA | PEG MW 400-10k | Inquiry |
BPG-1519 | Plys-PEG-Plys | PEG MW 1k-5k | Inquiry |
Currently, PEG hydrogels are widely used in the preparation of biological scaffolds to support the regeneration of tissues such as cartilage, skin, and heart. Their tunable physical and biological properties make them one of the core materials in the field of tissue engineering. As a smart material, PEG hydrogels can be chemically modified to achieve controlled drug release, playing a significant role in drug delivery systems. By integrating with 3D printing technology, drug carriers with complex internal structures can be printed for localized drug release. Furthermore, 3D bioprinting technology can embed cells into PEG hydrogels to simulate real tissue environments, promoting interactions between cells and scaffolds. Based on this, BOC Sciences provides high-quality PEG products and PEG solutions for 3D bioprinting applications, offering excellent biocompatibility, tunable mechanical properties, and photopolymerization capabilities. These solutions support cell culture, tissue engineering, and drug delivery, providing precise solutions for customized bioprinting.
What is 3D Bioprinting Used For?
Disruptive technology 3D bioprinting is already showing its broad value in many domains, including in the frontier of medicine, drug development, regenerative medicine, and tissue engineering. With bio-inks and high-precision printing, 3D bioprinting can build tissue and organ models in highly natural-looking shapes that solve the problem of traditional methods. Moreover, as technology keeps developing, its use is becoming wider to food manufacturing, cosmetics development, environmental engineering and basic science.
Tissue Engineering and Regenerative Medicine
The tissue engineering and regenerative medicine are some of the biggest use cases for 3D bioprinting. Print-off custom-made tissue replacements like skin, cartilage and bone using patients' own cells or other biocompatible substances. For burn patients or tissue damage repair, 3D printed tissues provide more exacting and tailored solutions. It's also used to build functional organ models, including liver and kidneys, establishing the path forward in organ transplantation.
Drug Development and Toxicity Testing
3D bioprinting technology is playing an increasingly important role in drug screening and toxicity testing. Compared to traditional 2D cell culture models, 3D printed tissue models are closer to the microenvironment of human tissues, providing more accurate predictions of drug efficacy and toxicity. For example, 3D printed liver models are widely used to evaluate the metabolic properties of new drugs, improving drug development efficiency and reducing the risk of failure.
Cancer Research and Personalized Medicine
Through 3D bioprinting, models of tumor microenvironments specific to individual patients can be created to study cancer progression mechanisms and the development of novel anti-cancer drugs. These models help researchers understand how tumors respond to different treatments, enabling the formulation of personalized medical plans. For example, organ tissues printed using the patient's own cells can reduce immune rejection, and printed skin tissues and bone scaffolds have shown significant results in clinical applications. Personalized scaffolds and implants created through this technology not only enhance repair outcomes but also meet complex medical needs.
** Recommended Products **
Cat. No. | Product Name | Molecular Weight | Price |
---|---|---|---|
BPG-0985 | 4-Arm PEG-Acrylate | MW 2k-20k | Inquiry |
BPG-1049 | 4-Arm PEG-OH | MW 500-20k | Inquiry |
BPG-4722 | 4-Arm PEG-NH2 | MW 2k-20k | Inquiry |
BPG-1033 | 4-Arm PEG-MAL | MW 2k-20k | Inquiry |
BPG-1087 | 4-Arm PEG-Thiol | MW 2k-20k | Inquiry |
BPG-1009 | 4-Arm PEG-DBCO | MW 5k-20k | Inquiry |
BPG-1105 | 8-Arm PEG-Acrylate | MW 10k-40k | Inquiry |
BPG-1150 | 8-Arm PEG-OH | MW 10k-40k | Inquiry |
BPG-1141 | 8-Arm PEG-NH2 | MW 10k-40k | Inquiry |
BPG-1138 | 8-Arm PEG-MAL | MW 10k-40k | Inquiry |
BPG-1171 | 8-Arm PEG-SH | MW 10k-40k | Inquiry |
BPG-1120 | 8-Arm PEG-DBCO | MW 10k-40k | Inquiry |
Reference
- Loukelis, K. et al. Advances in 3D bioprinting for regenerative medicine applications. Regen Biomater. 2024, 11: rbae033.
Why BOC Sciences?
-
Large Stock
More than 2000+ products in inventory
-
Global Delivery
Warehouses in multiple cities to ensure fast delivery
-
mg to kg
Multi specification for academic research and industrial production
-
24/7 Technical Support
Strict process parameter control to ensure product quality
Technical Support
- Aqueous Two-Phase System (ATPS) Technique
- Capillary Electrophoresis (CE) Technique
- Enzyme-linked immunosorbent assay (ELISA) Technique
- High performance liquid chromatography (HPLC) Technique
- Hydrophobic Interaction Chromatography (HIC) Technique
- PEGylated Protein Purification Techniques
- Radiolabeling Technique
- SDS-PAGE Technique
- Ultrafiltration Technique
Products
- Lipids
- PEG Derivatives by Structure
-
PEG Derivatives by Functional Group
- Acrylate/Acrylamide/Methacrylate PEG
- Aldehyde (Ald/CHO)PEG
- Alkyne PEG
- Amino PEG, PEG amine(-NH2)
- Azide PEG, Azido PEG(-N3)
- Biotin PEG
- Boc/Fmoc protected amine PEG
- Carboxylic Acid(-COOH) PEG
- Cholesterol PEG
- DBCO PEG
- DNP PEG
- DSPE PEG
- Epoxide glycidyl ether PEG
- FITC PEG
- Folate PEG
- Halide (chloride, bromide) PEG
- Hydrazide PEG
- Hydroxyl(-OH) PEG
- Maleimide(-MAL) PEG
- NHS ester PEG
- Nitrophenyl carbonate (NPC) PEG
- Norbornene PEG
- Olefin/Alkene/Vinyl PEG
- Orthopyridyl disulfide (OPSS) PEG
- Phosphate PEG
- Rhodamine PEG
- SCM PEG
- Silane PEG
- SPDP PEG
- Sulfonate (tosyl, mesyl, tresyl) PEG
- tert-Butyl protected carboxylate PEG
- Thiol(-SH) PEG
- Vinylsulfone PEG
- PEG Copolymers
-
PEG Raw Materials
- Small-molecule Polyethylene Glycol
- Polyethylene Glycol 1000
- Polyethylene Glycol 10000
- Polyethylene Glycol 1500
- Polyethylene Glycol 200
- Polyethylene Glycol 2000
- Polyethylene Glycol 20000
- Polyethylene Glycol 400
- Polyethylene Glycol 4000
- Polyethylene Glycol 600
- Polyethylene Glycol 6000
- Polyethylene Glycol 800
- Polyethylene Glycol 8000
Resources
-
Technical Information
- Aqueous Two-Phase System (ATPS) Technique
- Capillary Electrophoresis (CE) Technique
- Enzyme-linked immunosorbent assay (ELISA) Technique
- High performance liquid chromatography (HPLC) Technique
- How to Perform Polyethylene Glycol (PEG) Modification?
- Hydrophobic Interaction Chromatography (HIC) Technique
- Introduction of Polyethylene Glycol (PEG)
- Ion Exchange Chromatography (IEX) Technique
- PEG for Chemical Synthesis
- PEG for Cosmetic Application
- PEG for Drug Delivery
- PEG for Imaging Diagnosis
- PEG for Pharmaceutical Preparation
- PEG for Tissue Engineering
- PEG Purification Techniques of Plasmid DNA
- PEGylated Protein Purification Techniques
- Polyethylene Glycol (PEG) Modifier Selection Guide
- Radiolabeling Technique
- SDS-PAGE Technique
- Size Exclusion Chromatography (SEC) Technique
- Ultrafiltration Technique
-
Industry News
- Applications of PEG-DSPE: Drug Carriers and Drug Delivery
- Applications of Polyethylene Glycol (PEG) as Medical Devices
- Cholesterol: Definition, Structure, Synthesis, Types and Functions
- Classification of Lipid-Based Vaccine Adjuvants
- FDA approved PEGylated Products
- FDA-Approved Antibody-Drug Conjugates up to 2024
- How are Liposomes Different from Micelles?
- How Lipid Nanoparticles (LNPs) Deliver RNA Drugs?
- Hyaluronic Acid & PEGylated Hyaluronic Acid
- Ionizable Lipids for RNA Delivery
- Lipid Classification and Drug Delivery Systems
- Lipid Formulations: Key Absorption-Enhancing Technologies in New Drug Development
- Lipid-Drug Conjugates (LDCs) for Nanoparticle Drug Delivery
- Liposome in Drug Delivery
- Overview of Liposome Preparation Process
- PEG in Pharmaceutical Preparations (I): Solvents, Lubricants, Adhesives and More
- PEG in Pharmaceutical Preparations (II): Stabilizers, Plasticizers and Modification Materials
- PEG Linkers in Antibody Drug Conjugates and PROTACs
- PEG-DSPE Block Copolymers and Their Derivatives
- PEGylated Drugs: Definition, Structure, Classification and Benefits
- PEGylated RGD Peptides: A Promising Tool for Targeted Drug Delivery
- Pharmacokinetics and Bioanalysis of PEGylated Drugs
- Polyethylene Glycol (PEG) Modified Targeting Nanomaterials
- Preparation Method of PEG Hydrogel
- The PROTAC Technology in Drug Development
- Vaccines: Definition, History, Ingredients, Types and Mechanism of Action
- What are Lipid Excipients and Their Applications?
- What are Lipid Nanoparticles and Their Applications?
- What are Lipid-Drug Conjugates (LDCs)?
- What are Lipids?
- What are Monodispersed and Polydispersed PEGs?
- What are PEG Lipids?
- What are Phospholipids?
- What are Sterols? - Definition, Structure, Function, Examples and Uses
- What is Biotinylation and Biotinylated PEG?
- What is Click Chemistry?
- What is Hydrogel?
- What is Methoxy Polyethylene Glycol (mPEG)?
- What is Nanogels and Its Applications?
- What is the Formulation of Lipid Nanoparticles (LNPs)?
Our Feature
BOC Sciences supplies a unique variety of PEG derivatives and functional PEG polymers. Our products offer the most diverse collection of reactivity, ready-to-use functionality, and molecular weight options that you will not find anywhere else.
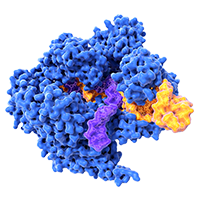
PEGylation of Peptides
and Proteins
Reduce the Immunogenicity of Peptide/Protein Drugs
Learn More
APPLICATIONS
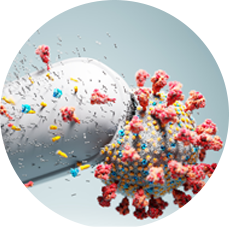
PEG linkers For Drug
Improved Circulation Half-Life
Learn More
Have Customer
Reviewed On Us?
Chat With Us