Liposomes vs. Lipid Nanoparticles: What are the Differences?
Since the discovery of liposomes in the 1960s, they have played an important role in the field of drug delivery. With the introduction of nanotechnology, lipid nanoparticles have been widely used to deliver various types of drugs. Following the outbreak of the COVID-19 pandemic, lipid nanoparticles have played a crucial role in the development of COVID-19 vaccines by protecting and delivering mRNA. They also serve an important delivery role in therapeutic areas and are extensively used in medical imaging, nutrition, cosmetics, agriculture, and nano-micro reactors.
What are Liposomes?
Liposomes were first discovered in 1961 by scientists Alec Douglas Bangham and R. W. Horne under a microscope. Liposomes are vesicular structures composed of lipid molecules, with their lipid bilayer forming a hydrophobic shell surrounding an aqueous interior cavity, exhibiting both hydrophilic and hydrophobic properties. Liposomes range in size from 20 nm to 1000 nm, where hydrophilic drugs can be encapsulated in the aqueous interior, while hydrophobic drugs can be incorporated within the hydrocarbon chain region of the lipid bilayer. Liposomes can stabilize therapeutic compounds and overcome barriers to cellular and tissue uptake, enhancing the targeting of compounds to disease sites and reducing accumulation in non-target organs. Depending on the route of administration and disease location, liposomes can further improve the delivery of encapsulated compounds in conjunction with different delivery platforms.
Fig. 1. Liposome structure.
Liposomes used for drug delivery are primarily composed of various phospholipids and cholesterol, with phospholipids (glycerophospholipids and sphingolipids) serving as the basic framework. Glycerophospholipids are lipids with glycerol as their backbone, while sphingolipids have sphingosine as their backbone. Cholesterol is an amphipathic neutral lipid that can regulate the fluidity of the lipid bilayer, increasing the stability of liposomes and reducing drug leakage, thereby prolonging and controlling drug release. In addition to phospholipids and cholesterol, liposomes can also incorporate polysaccharides (such as oligosaccharides, chitosan, and hyaluronic acid) and surfactants to enhance their stability and regulate drug release.
Liposomal Benefits
Based on different structures and properties, liposomes can be classified into unilamellar liposomes, multilamellar liposomes, and multivesicular liposomes, among others. Additionally, based on the charge of the liposomes, they can be divided into cationic liposomes (such as DOTAP), anionic liposomes (such as DOPS), and neutral liposomes (such as DSPE). There are also functional types, including long-circulating liposomes, glycosylated liposomes, thermosensitive liposomes, pH-sensitive liposomes, immunoliposomes, magnetic liposomes, and elastic liposomes. Structurally similar to biological membranes, liposomes exhibit excellent biocompatibility. As drug carriers, liposomes have the following advantages:
- Ability to Carry Different Types of Drugs/Genes: The unique bilayer structure of liposomes allows for the encapsulation and protection of various types of drugs and genes, including hydrophilic and lipophilic drugs.
- Potential for Multiple Routes of Administration: Liposomes can be administered through various routes, including oral, injection, and topical application, allowing for the selection of the most suitable route based on specific needs.
- Prevention of Chemical and Biological Degradation: Due to their structural similarity to cell membranes, liposomes can protect drugs from chemical and biological degradation, prolonging their half-life and improving stability and bioavailability.
- Reduction of Non-Specific Side Effects and Toxicity: By encapsulating drugs within, liposomes minimize the impact of drugs on non-target tissues, reducing non-specific side effects and toxicity while enhancing drug efficacy and therapeutic outcomes.
- Multifunctional Chemical Modifications and Targeting Ability: Liposomes can undergo chemical modifications, allowing for the attachment of specific ligands or functional groups to achieve targeted effects, thereby improving drug targeting and selectivity.
- Compatibility with Biodegradable, Non-Toxic Materials: Liposomes can be compatible with biodegradable and non-toxic materials, reducing adverse effects on the human body.
What is Liposomal Used For?
Liposomes, the first generation of lipid nanoparticles, have successfully transitioned from concept to clinical application, serving as multifunctional carriers for various drugs, including small molecules, proteins, and nucleic acids. They have been launched for indications like anti-tumor and analgesic therapies and are also used in ocular drug delivery, antimalarial treatments, and vaccine preparations, including influenza vaccines.
- Their primary role in drug delivery is in cancer treatment, targeting tumor tissues through their ability to penetrate permeable blood vessels. Liposomes can be modified with polymers and ligands, enhancing stability and targeting. They carry drugs with diverse physicochemical properties, making them ideal for nanomedicine. Notable cancer treatments include Marqibo® (Vincristine), Taxol® (Paclitaxel), and Onivyde® (Irinotecan).
- In antifungal therapy, liposomes address the challenge of biofilms that hinder drug uptake, particularly in immunocompromised patients. Their biocompatibility reduces toxicity and allows efficient drug delivery. Current antifungal options include Amphotericin B liposomes like Ambisome®, Abelcet®, and Amphotec®.
Lipids from BOC Sciences
BOC Sciences is at the forefront of providing high-quality lipid products for liposomes and lipid nanoparticles, catering to the diverse needs of the pharmaceutical and biotechnology industries. Our extensive portfolio includes various lipid compounds, such as phospholipids, sphingolipids, and lipid derivatives, essential for the formulation and production of liposomes and lipid nanoparticles.
Cat. No. | Product Name | CAS | Inquiry |
---|---|---|---|
BPG-3281 | 27-Hydroxycholesterol | 20380-11-4 | Inquiry |
BPG-3282 | 7α-Hydroxy Cholesterol | 566-26-7 | Inquiry |
BPG-3283 | 22-alpha-Hydroxy Cholesterol | 17954-98-2 | Inquiry |
BPG-3614 | C13-112-tri-tail | 1381861-96-6 | Inquiry |
BPG-3615 | C13-113-tri-tail | 1381861-86-4 | Inquiry |
BPG-3284 | 7β-Hydroxy Cholesterol | 566-27-8 | Inquiry |
BPG-2972 | DSPE-PEG-COOH sodium salt | 1403744-37-5 | Inquiry |
BPG-2971 | DSPE-PEG (2000) Biotin | 385437-57-0 | Inquiry |
What are Lipid Nanoparticles?
Lipid Nanoparticles (LNPs) are nano-sized particles formed using lipids, consisting of lipid vesicles with a uniform lipid core, essentially representing solid lipid particles. These lipid nanoparticle carriers utilize specific lipids as wall materials to encapsulate or adsorb active ingredients. Generally, these lipids exhibit good biocompatibility, do not induce allergic or immunosuppressive reactions, and effectively protect the stability of the contents. They can be directed towards specific targets, providing targeting capabilities that enhance the application efficiency of active substances. Therefore, this technology is widely used for the delivery of small molecules and nucleic acid drugs.
Fig. 2. Lipid nanoparticle structure.
Typically, lipid nanoparticle carriers have a particle size ranging from 50 to 1000 nm. Conventional lipid particle carriers, due to their larger size, exhibit poor cellular permeability, and larger particle sizes can lead to structural instability. Lipid nanoparticle carriers effectively resolve these issues; they possess physicochemical properties similar to conventional lipid particle carriers while significantly improving stability, absorption, biodistribution, and bioavailability compared to traditional lipid carriers. The very small size of lipid nanoparticle carriers greatly enhances the tissue permeability of the carriers. This provides active substances with good in vivo targeting capabilities. Furthermore, reducing particle size increases the specific surface area. Within the same volume, the increased surface area allows for more active ingredients to be loaded. The increased specific surface area also significantly enhances the contact area, facilitating more interactions between these active substances and their target, thus improving efficacy. This allows for lower doses to achieve the same therapeutic effect.
Lipid Nanoparticle Formulation
Lipid nanoparticles are spherical vesicles composed of one (unilamellar) or multiple (multilamellar) phospholipid bilayers, typically made up of four components: cationic lipids, helper lipids, cholesterol, and pegylated lipids (PEG-lipids). With the development of various lipid nanoparticles, they now include not only liposomes but also solid lipid nanoparticles (SLN), nanostructured lipid carriers (NLC), cationic LNPs, targeted liposomes, stealth liposomes, and cubosomes, among others.
Types | Features | Applications |
---|---|---|
Liposomes | Hydrophilic drugs are encapsulated in the inner aqueous phase; lipophilic drugs are encapsulated in the lipid layer. | The earliest lipid nanoparticles are suitable for various drug and gene therapies. |
Solid lipid nanoparticles/nanostructured lipid carriers | High physical stability; high loading capacity and bioavailability; reduced fluidity to control drug release. | Process scaling and production are simpler. |
Cationic lipid nanoparticles | Stabilizes nucleic acids; extends the retention time against nuclease degradation. | Widely used in non-viral vector systems for nucleic acid drug delivery. |
Targeted liposomes | Surface ligands precisely target cell receptors | Improves efficacy. |
Stealth liposomes | Avoid recognition by the immune system; increase circulation half-life; promote passive accumulation of drugs | PEGylation technology, developed from egg-derived drugs, can avoid immune responses. |
Cubic liposomes | Good stability; controllable core size; capable of containing active lipids. | Can be used in drug delivery systems, membrane bioreactors, and biosensors. |
Table 1. Characteristics and applications of various lipid nanoparticles.
Lipid Nanoparticles Synthesis
With the development of technology, the preparation techniques for lipid nanoparticles have also advanced. Initially, researchers only used solid lipids to construct lipid nanoparticles, known as solid lipid nanoparticles (SLN). However, as their application expanded, SLNs revealed issues such as low active ingredient loading capacity, lipid particle aggregation, leakage of active ingredients, and poor stability of sustained-release functions. In response, researchers began to incorporate a certain amount of liquid oils into the solid lipid wall materials to improve their carrier functionality, leading to the development of the second generation of lipid particles, known as nanostructured lipid carriers (NLC). The addition of liquid oils in NLC disrupts the ordered crystalline structure of the original solid lipid wall material, resulting in an increased loading of active ingredients and enhancing the stability of the crystalline structure, making it less prone to leakage.
Traditional preparation techniques for conventional lipid particle carriers, such as the ethanol injection method, thin film dispersion method, reverse evaporation method, and freeze-thaw method, can effectively produce conventional lipid particle carriers. However, the average particle size of the obtained lipid particles is relatively large, and the encapsulation rate is low. To achieve the required particle size for lipid nanoparticles, researchers have developed many emerging preparation technologies, including microfluidic mixing technology, dynamic high-pressure micro-jet technology, high-pressure homogenization, and supercritical carbon dioxide fluid technology. In comparison, these new lipid nanoparticle carrier preparation technologies are more effective at producing small-sized nano lipid particles while offering high encapsulation rates, simple process equipment, mild processing conditions, and fewer limitations for industrial scale-up production. Among these, microfluidic mixing technology is currently a commonly used preparation method; it is relatively simple and quick, operates under mild conditions, and can be easily scaled up for production.
Applications of Lipid Nanoparticles
mRNA Vaccine Delivery
In recent years, lipid nanoparticles (LNPs) have successfully emerged as a delivery platform for RNA vaccines and therapies. Naked RNA is a negatively charged, hydrophilic macromolecule that faces challenges in entering cells due to electrostatic repulsion from cell membranes and rapid degradation by ubiquitous ribonucleases. Therefore, a protective shell is necessary for RNA to penetrate cells. Since cell membranes are primarily composed of lipids, encapsulating RNA within lipid vesicles can facilitate its passage through the cell membrane and release RNA into the cytoplasm. Consequently, the vesicles should initially contain positively charged lipids that can interact with negatively charged RNA. However, vesicles composed of permanently cationic lipids may cause cytotoxicity due to electrostatic interactions with the negatively charged cell membrane. Thus, lipid structures have evolved into molecules that respond to the acidic environment of endosomes, acquiring a positive charge.
Tumor Therapy
Tumor cells exhibit strong invasive capabilities, significant destructive potential, and a high resemblance to normal cells, making treatment challenging. The field of anti-tumor research has long been a key focus in the pharmaceutical industry. Liposomes, with their unique advantages, have demonstrated tremendous potential in cancer treatment. Over the years, with the deepening of research, the therapeutic approaches and methods utilizing liposomes as carriers for anti-tumor drugs have become increasingly widespread, forming a developmental process characterized by distinct phases.
Anti-Inflammatory and Anti-Infection Drug Carriers
Taking tuberculosis treatment as an example, rifampicin, the first-line drug for treating pulmonary tuberculosis, can be formulated into rifampicin liposome aerosols. Administered via the lungs, this formulation allows for uniform deposition of the drug in the pulmonary region, increasing the retention of the drug in the lungs, prolonging the drug release process, and preventing local irritation, thereby enhancing efficacy and reducing extrathoracic side effects.
Treatment of Neurodegenerative Diseases
For chronic neurodegenerative diseases in adults, such as Alzheimer's and Parkinson's disease, delivering drugs across the blood-brain barrier (BBB) presents a significant challenge. However, lipid vesicles modified with BBB-targeting ligands can cross the BBB, achieving therapeutic effects for drugs aimed at treating Alzheimer's and Parkinson's diseases.
Anti-Malaria
Anti-malarial drugs often suffer from poor water solubility, low permeability, and low bioavailability, coupled with the non-specific targeting of intracellular parasites, resulting in high dosage requirements and significant side effects. Liposome technology can effectively address these issues, achieving sustained and controlled release while reducing the progression of drug resistance and minimizing adverse reactions.
Liposome vs Lipid Nanoparticle
The key differences between liposomes and lipid nanoparticles lie not only in their respective applications but also in their morphological structures, compositions, and production processes:
Fig. 3. Liposome vs lipid nanoparticle (Nat Rev Drug Discov. 2021, 20(2): 101-124).
- Broadly defined, lipid nanoparticles are nanoscale particles formed from lipids, which means that liposomes can also be categorized as a type of lipid nanoparticle. However, in specific scientific research, lipid nanoparticles refer to a particular type of nanoparticle that is distinct from liposomes. The primary distinction between liposomes and lipid nanoparticles is in their morphology: liposomes consist of a bilayer of lipids arranged in an ordered manner, forming closed vesicles with a hydrophilic internal cavity, while lipid nanoparticles lack a hydrophilic cavity. Instead, lipid nanoparticles contain cationic phospholipids and negatively charged nucleic acids that electrostatically interact within their interior, forming a multilayer core dispersed among the lipid layers.
- Regarding composition, the main components are roughly similar, with both containing lipids and cholesterol; however, lipid nanoparticles must include ionizable lipids, whereas liposomes do not have strict requirements for lipid types. There are significant differences in the proportions of various components between liposomes and lipid nanoparticles, particularly in the amount of cholesterol. For example, in the classic liposome product DOXIL, the ratio is HSPC:CHOL:DSPE-PEG2000 = 3:1:1, while in the two commercially available mRNA COVID-19 vaccines, cholesterol comprises 42.7% (Pfizer/BioNTech) and 38.5% (Moderna), significantly higher than the cholesterol content in liposomes.
- In terms of production processes, the upstream procedures and steps for liposomes and lipid nanoparticles differ, but the downstream production processes are nearly identical. The traditional liposome preparation process begins with the formation of coarse liposomes from lipid and aqueous phases, followed by homogenization or extrusion to control particle size within a specific range. In contrast, lipid nanoparticles are produced by rapidly mixing lipid ethanol solutions with nucleic acid acidic aqueous solutions using a microfluidic mixing system, where the connector of the microfluidic mixing system can control particle size during the collision of the two phases. The downstream buffer exchange processes for liposomes and lipid nanoparticles are nearly the same, utilizing tangential flow filtration (TFF) technology for buffer exchange or purification, followed by terminal sterilization through a 0.22 μm filter.
In Conclusion
Liposomes and lipid nanoparticles share the same origin. Liposomes have played an important role in the field of drug delivery, involving numerous indications, while lipid nanoparticles are currently used only for the delivery of nucleic acid substances. With the advancement of related technologies, it is believed that liposomes and lipid nanoparticles will assist more drugs in achieving efficient delivery. Based on this, BOC Sciences, in addition to providing a wide range of lipid products, also supports liposome preparation and lipid nanoparticle development services. Our expertise in lipid chemistry enables us to customize lipid formulations suitable for specific applications, including cancer treatment, vaccine delivery and antifungal treatment.
** Recommended Products **
Catalog | Name | CAS | Price |
---|---|---|---|
BPG-3010 | Monophosphoryl Lipid A | 1246298-63-4 | Inquiry |
BPG-3287 | 25-Hydroxy-Cholesterol | 2140-46-7 | Inquiry |
BPG-3312 | 7Keto-25-hydroxy cholesterol | 64907-23-9 | Inquiry |
BPG-3623 | MHAPC-Chol | 1027801-74-6 | Inquiry |
BPG-3624 | DMHAPC-Chol | 794494-38-5 | Inquiry |
BPG-3626 | Cholesteryl chloroformate | 7144-08-3 | Inquiry |
BPG-3659 | AA3-DLin | 2832061-33-1 | Inquiry |
BPG-3682 | 113-O12B | 2803699-72-9 | Inquiry |
BPG-3706 | BP Lipid 113 | 2714482-23-0 | Inquiry |
BPG-3765 | 1-Azidodecane | 62103-13-3 | Inquiry |
BPG-3766 | Dodecyl azide | 13733-78-3 | Inquiry |
BPG-3768 | 7-methyl-8-oxononanoic acid | 407627-97-8 | Inquiry |
BPG-3785 | heptyl 8-bromooctanoate | 612842-05-4 | Inquiry |
BPG-3786 | Undecyl 8-bromooctanoate | 2707440-34-2 | Inquiry |
BPG-3800 | heptadecan-9-yl 10-bromodecanoate | 2761458-99-3 | Inquiry |
BPG-3802 | 6-bromohexyl 2-hexyldecanoate | 2644752-91-8 | Inquiry |
BPG-3847 | Isoleukotoxin Diol | 73889-55-1 | Inquiry |
BPG-3851 | Linoleyl methane sulfonate | 51154-39-3 | Inquiry |
BPG-3873 | 2-undecyloxirane | 1713-31-1 | Inquiry |
Reference
- Mitchell, M.J. et al. Engineering precision nanoparticles for drug delivery. Nat Rev Drug Discov. 2021, 20(2): 101-124.
Why BOC Sciences?
-
Large Stock
More than 2000+ products in inventory
-
Global Delivery
Warehouses in multiple cities to ensure fast delivery
-
mg to kg
Multi specification for academic research and industrial production
-
24/7 Technical Support
Strict process parameter control to ensure product quality
Technical Support
- Aqueous Two-Phase System (ATPS) Technique
- Capillary Electrophoresis (CE) Technique
- Enzyme-linked immunosorbent assay (ELISA) Technique
- High performance liquid chromatography (HPLC) Technique
- Hydrophobic Interaction Chromatography (HIC) Technique
- PEGylated Protein Purification Techniques
- Radiolabeling Technique
- SDS-PAGE Technique
- Ultrafiltration Technique
Products
- Lipids
- PEG Derivatives by Structure
-
PEG Derivatives by Functional Group
- Acrylate/Acrylamide/Methacrylate PEG
- Aldehyde (Ald/CHO)PEG
- Alkyne PEG
- Amino PEG, PEG amine(-NH2)
- Azide PEG, Azido PEG(-N3)
- Biotin PEG
- Boc/Fmoc protected amine PEG
- Carboxylic Acid(-COOH) PEG
- Cholesterol PEG
- DBCO PEG
- DNP PEG
- DSPE PEG
- Epoxide glycidyl ether PEG
- FITC PEG
- Folate PEG
- Halide (chloride, bromide) PEG
- Hydrazide PEG
- Hydroxyl(-OH) PEG
- Maleimide(-MAL) PEG
- NHS ester PEG
- Nitrophenyl carbonate (NPC) PEG
- Norbornene PEG
- Olefin/Alkene/Vinyl PEG
- Orthopyridyl disulfide (OPSS) PEG
- Phosphate PEG
- Rhodamine PEG
- SCM PEG
- Silane PEG
- SPDP PEG
- Sulfonate (tosyl, mesyl, tresyl) PEG
- tert-Butyl protected carboxylate PEG
- Thiol(-SH) PEG
- Vinylsulfone PEG
- PEG Copolymers
-
PEG Raw Materials
- Small-molecule Polyethylene Glycol
- Polyethylene Glycol 1000
- Polyethylene Glycol 10000
- Polyethylene Glycol 1500
- Polyethylene Glycol 200
- Polyethylene Glycol 2000
- Polyethylene Glycol 20000
- Polyethylene Glycol 400
- Polyethylene Glycol 4000
- Polyethylene Glycol 600
- Polyethylene Glycol 6000
- Polyethylene Glycol 800
- Polyethylene Glycol 8000
Resources
-
Technical Information
- Aqueous Two-Phase System (ATPS) Technique
- Capillary Electrophoresis (CE) Technique
- Enzyme-linked immunosorbent assay (ELISA) Technique
- High performance liquid chromatography (HPLC) Technique
- How to Perform Polyethylene Glycol (PEG) Modification?
- Hydrophobic Interaction Chromatography (HIC) Technique
- Introduction of Polyethylene Glycol (PEG)
- Ion Exchange Chromatography (IEX) Technique
- PEG for Chemical Synthesis
- PEG for Cosmetic Application
- PEG for Drug Delivery
- PEG for Imaging Diagnosis
- PEG for Pharmaceutical Preparation
- PEG for Tissue Engineering
- PEG Purification Techniques of Plasmid DNA
- PEGylated Protein Purification Techniques
- Polyethylene Glycol (PEG) Modifier Selection Guide
- Radiolabeling Technique
- SDS-PAGE Technique
- Size Exclusion Chromatography (SEC) Technique
- Ultrafiltration Technique
-
Industry News
- Applications of PEG-DSPE: Drug Carriers and Drug Delivery
- Applications of Polyethylene Glycol (PEG) as Medical Devices
- Cholesterol: Definition, Structure, Synthesis, Types and Functions
- Classification of Lipid-Based Vaccine Adjuvants
- FDA approved PEGylated Products
- FDA-Approved Antibody-Drug Conjugates up to 2024
- How are Liposomes Different from Micelles?
- How Lipid Nanoparticles (LNPs) Deliver RNA Drugs?
- Hyaluronic Acid & PEGylated Hyaluronic Acid
- Ionizable Lipids for RNA Delivery
- Lipid Classification and Drug Delivery Systems
- Lipid Formulations: Key Absorption-Enhancing Technologies in New Drug Development
- Lipid-Drug Conjugates (LDCs) for Nanoparticle Drug Delivery
- Liposome in Drug Delivery
- Overview of Liposome Preparation Process
- PEG in Pharmaceutical Preparations (I): Solvents, Lubricants, Adhesives and More
- PEG in Pharmaceutical Preparations (II): Stabilizers, Plasticizers and Modification Materials
- PEG Linkers in Antibody Drug Conjugates and PROTACs
- PEG-DSPE Block Copolymers and Their Derivatives
- PEGylated Drugs: Definition, Structure, Classification and Benefits
- PEGylated RGD Peptides: A Promising Tool for Targeted Drug Delivery
- Pharmacokinetics and Bioanalysis of PEGylated Drugs
- Polyethylene Glycol (PEG) Modified Targeting Nanomaterials
- Preparation Method of PEG Hydrogel
- The PROTAC Technology in Drug Development
- Vaccines: Definition, History, Ingredients, Types and Mechanism of Action
- What are Lipid Excipients and Their Applications?
- What are Lipid Nanoparticles and Their Applications?
- What are Lipid-Drug Conjugates (LDCs)?
- What are Lipids?
- What are Monodispersed and Polydispersed PEGs?
- What are PEG Lipids?
- What are Phospholipids?
- What are Sterols? - Definition, Structure, Function, Examples and Uses
- What is Biotinylation and Biotinylated PEG?
- What is Click Chemistry?
- What is Hydrogel?
- What is Methoxy Polyethylene Glycol (mPEG)?
- What is Nanogels and Its Applications?
- What is the Formulation of Lipid Nanoparticles (LNPs)?
Our Feature
BOC Sciences supplies a unique variety of PEG derivatives and functional PEG polymers. Our products offer the most diverse collection of reactivity, ready-to-use functionality, and molecular weight options that you will not find anywhere else.
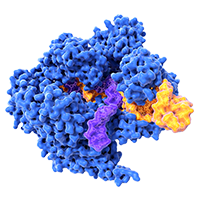
PEGylation of Peptides
and Proteins
Reduce the Immunogenicity of Peptide/Protein Drugs
Learn More
APPLICATIONS
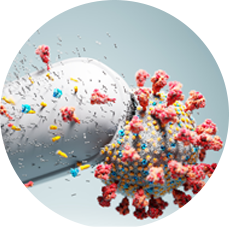
PEG linkers For Drug
Improved Circulation Half-Life
Learn More
Have Customer
Reviewed On Us?
Chat With Us