Lipid Nanoparticles for Gene Delivery
Lipid nanoparticles (LNPs) have emerged as a groundbreaking technology in the field of gene delivery, owing to their exceptional biocompatibility, tunability, and highly efficient nucleic acid delivery capabilities. LNPs are typically composed of ionizable lipids, helper lipids, cholesterol derivatives, and PEG-lipids. By optimizing these components, LNPs can achieve efficient encapsulation and delivery of various nucleic acid molecules, including mRNA, siRNA, and DNA. Compared to traditional viral vectors, LNPs offer significant advantages such as reduced immunogenicity and enhanced safety. Furthermore, their production processes are scalable, making them widely applicable in emerging gene therapies.
What is Gene Therapy?
Gene therapy is an advanced medical technology that uses gene-editing tools to modify human genetic information, aiming to treat a wide range of hereditary and non-hereditary diseases. The human genome consists of approximately 20,000 genes distributed across 23 pairs of chromosomes. Each gene carries specific genetic information that, through the processes of DNA replication and transcription, is converted into RNA and subsequently into proteins, regulating various biological functions. Many hereditary diseases, such as cystic fibrosis, sickle cell disease, and sickle cell anemia, result from gene mutations. Through gene editing, scientists can precisely repair these mutated genes, potentially curing these conditions. Additionally, gene editing can be used in cancer treatment by modifying cancer cell genes to inhibit their growth, offering a promising therapeutic approach.
FDA Approved Gene Therapies
Gene Therapy Based on CD34+ HSC | ||
---|---|---|
Skysona (2022) | Autologous CD34+ hematopoietic stem cells (HSCs) modified using the Lenti-D vector to express the ABCD1 gene. | For patients with a cerebral form of adrenoleukodystrophy. |
Zynteglo (2022) | Autologous CD34+ HSCs modified with BB305 lentiviral vector encoding the β-globin gene (βA-T87Q-globin gene). | For adults and children with certain types of β-thalassemia. |
Carvykti (2023) | Autologous CD34+ HSCs modified via electroporation of CRISPR/Cas9 RNP complexes to reduce BCL11A, increasing fetal hemoglobin production. | For patients aged 12 and older with certain forms of sickle cell disease. |
Lyfgenia (2023) | Autologous CD34+ HSCs modified with BB305 lentiviral vector encoding the β-globin gene (βA-T87Q-globin gene). | For patients aged 12 and older with specific types of sickle cell disease. |
Libmeldy (2024) | Autologous CD34+ HSCs modified with a lentiviral vector encoding human arylsulfatase A. | For children with certain forms of metachromatic leukodystrophy (MLD). |
Adenovirus/Adeno-Associated Virus-Based Gene Therapies | ||
Luxturna (2017) | A recombinant adeno-associated virus (AAV2) containing a transgene encoding RPE65. | For patients with retinal dystrophy associated with RPE65 mutations. |
Zolgensma (2019) | A recombinant adeno-associated virus (AAV9) containing a transgene encoding the human survival motor neuron (SMN) protein. | For pediatric patients with certain types of spinal muscular atrophy. |
Dostarlimab (2022) | A recombinant adenovirus containing a transgene encoding human interferon alpha-2b (IFNα2b). | For adult patients with certain types of bladder cancer. |
Hemgenix (2022) | A recombinant adeno-associated virus (AAV) containing a transgene encoding the gain-of-function Padua variant (R338L) of human Factor IX. | For adult patients with certain types of hemophilia B. |
Elevidys (2023) | A recombinant adeno-associated virus (AAVrh74) containing a transgene encoding microdystrophin. | For pediatric patients with certain types of Duchenne muscular dystrophy. |
Roctavian (2023) | A recombinant adeno-associated virus (AAV5) containing a transgene encoding the SQ form of human coagulation Factor VIII with B-domain deletion (hFVIII-SQ). | For adult patients with certain types of hemophilia A. |
Oncolytic Virus-Based Gene Therapies | ||
Imlygic (2015) | A recombinant HSV-1 expressing human GM-CSF to promote antitumor immune responses. | For the local treatment of certain unresectable melanoma lesions. |
Vyjuvek (2023) | A recombinant HSV-1 expressing the type VII collagen gene (COL7A1). | For the local treatment of specific wounds in patients with dystrophic epidermolysis bullosa caused by genetic mutations. |
How Does Gene Therapy Work?
The basic principle of gene therapy is to correct or compensate for diseases caused by genetic defects or mutations by introducing normal or therapeutic genes into human cells. These genes can be delivered into human cells through viral vectors, non-viral vectors, or gene editing technologies, thereby producing the corresponding gene expression products. Gene therapy can be divided into two main categories based on the target cells: somatic gene therapy and germline gene therapy. Somatic gene therapy mainly targets the treatment of already-formed diseases, while germline gene therapy attempts to prevent genetic diseases in future generations by modifying the genes of reproductive cells. Among these, CRISPR-Cas9 technology is the most widely used gene editing tool, as it can precisely cut DNA and insert new gene sequences at the break points. The main steps of gene therapy include the following stages:
- Selection and Design of Target Genes: Based on the disease pathology, target genes are chosen for therapeutic effects, such as repairing mutated genes, expressing functional proteins, or producing short interfering RNAs (siRNAs) to suppress disease-causing genes.
- Construction of Delivery Vectors: Foreign genes must be delivered into the patient using vectors, which can be viral or non-viral systems. These vectors are designed to ensure high efficiency, specificity, and safety.
- Gene Transfer and Integration: The vector delivers the target gene into specific cells, where the gene may integrate into the host genome or express its function without integration.
- Gene Expression Regulation and Therapeutic Effect: The foreign gene expresses therapeutic proteins or regulates signaling pathways to intervene in disease progression.
Gene Delivery
Gene delivery is a core technology in gene therapy, aiming to deliver functional exogenous genes precisely and safely to target cells while ensuring their effective expression. This process must overcome multiple biological barriers, such as nucleic acid degradation, crossing cell membranes, and maintaining gene stability and expression efficiency within cells. Thus, advancements in gene delivery technologies are pivotal to the success of gene therapy. Gene delivery techniques are mainly categorized into viral vectors and non-viral vectors, incorporating advanced targeting technologies:
Viral Vectors
Derived from viruses such as adenoviruses, adeno-associated viruses (AAV), retroviruses, or lentiviruses. Scientists remove viral genes and modify these vectors to carry only therapeutic genes, leveraging viruses' natural mechanisms to enter cells. While viral vectors are efficient, they pose potential safety risks, such as triggering immune responses or causing insertional mutagenesis (unexpected integration into the host genome).
Non-Viral Vectors
Alternatives that do not use viruses, including plasmids (circular DNA molecules), liposomes, polymers, or nanoparticles. These vectors generally have a lower risk of insertional mutagenesis but may have lower delivery efficiency compared to viral vectors. Nanoparticle systems utilize polymers (e.g., polyethylene glycol, lipids, polylactic-co-glycolic acid) or inorganic materials (e.g., gold nanoparticles) to encapsulate genes into stable delivery vehicles. These particles can be modified for biocompatibility, targeting capabilities, and controlled release, making them an essential research focus in non-viral delivery technologies.
Gene Delivery Nanoparticles
Lipid nanoparticles have gained renewed attention as gene delivery vectors, especially with their use in COVID-19 mRNA vaccines. LNPs used in gene therapy are typically composed of ionizable cationic lipids, phospholipids, cholesterol, and PEG-lipids. Structurally, they feature a solid or oily core, contrasting with traditional single-layer liposomes characterized by a lipid bilayer and an aqueous pool in the center. Since liposomes were approved early on by regulatory agencies for clinical small-molecule drugs, they were quickly identified as potential delivery vehicles for gene therapy. Insights gained from liposomal drug delivery have significantly accelerated the development of LNPs for gene, RNAi, mRNA, and CRISPR delivery. For example, in 2018, the FDA approved the first LNP-based gene therapy drug—siRNA patisiran (Onpattro; Alnylam)—for treating hereditary transthyretin-mediated amyloidosis-induced polyneuropathy.
Fig. 2. Lipid nanoparticles for gene delivery (Adv Genet. 2014, 88: 13-36).
Gene therapies can be classified into four main categories based on their potential mechanisms of action: 1) Gene addition or replacement; for example, viral vectors containing specific genes or genes encoded in mRNA encapsulated in LNPs. 2) Gene expression control; for example, siRNA encapsulated in LNPs or siRNA conjugated with the hepatocyte-targeting ligand GalNac (N-acetylgalactosamine). 3) Gene editing; for example, viral vectors or LNPs delivering components of the CRISPR-Cas9 gene editing system. 4) DNA or RNA vaccines; for example, mRNA vaccines encapsulated in LNPs or polymer nanoparticles. Among these four categories of gene drugs, LNPs have an average permeability (percentage of assets using this modality) of 7%. This is the highest for nucleic acid-based vaccines and gene editing, reflecting that the nucleic acids to be delivered in these fragments are typically too large for GalNAc conjugation. LNPs have lower permeability in gene addition or replacement, as adeno-associated virus (AAV)-based delivery provides greater utility. Gene expression control has the lowest permeability due to the competition with GalNAc conjugation. Overall, LNP-driven genomic drug pipelines are primarily focused on DNA and RNA vaccines, mostly in Phase I trials.
Lipid Nanoparticles Synthesis
BOC Sciences specializes in providing comprehensive solutions for the development and production of lipid nanoparticles. We supply high-quality key materials, including lipids, excipients, and functional molecules, ensuring the efficiency and stability of LNP formulations. Additionally, we offer customized development services covering formulation design, process optimization, and scale-up production to meet diverse needs in drug delivery, gene therapy, and vaccine development. Leveraging advanced technology platforms and a professional team, we achieve precise material characterization and quality control, ensuring consistent performance in every batch. BOC Sciences is committed to being your reliable partner in LNP development, accelerating your innovation journey.
Catalog | Name | CAS | Price |
---|---|---|---|
BPG-3010 | Monophosphoryl Lipid A | 1246298-63-4 | Inquiry |
BPG-3011 | Trehalose-6,6'-dibehenate | 66758-35-8 | Inquiry |
BPG-3016 | 3A-MPLA | 1699735-80-2 | Inquiry |
BPG-3017 | 4A-MPLA | 2260669-09-6 | Inquiry |
BPG-3025 | 16:0 DBCO PE Ammonium salt | 2260670-42-4 | Inquiry |
BPG-3026 | 16:0(alkyne)-18:1 PE | 2260670-76-4 | Inquiry |
BPG-3027 | Oleic Acid (18-azido) | 2260670-77-5 | Inquiry |
BPG-3032 | 18:1 propargyl PC | 1830366-41-0 | Inquiry |
Why Use Lipid Nanoparticles?
LNPs offer significant advantages in gene therapy due to their long-standing history of development, low toxicity, and low immunogenicity. The industry's extensive experience in large-scale production of liposomes also makes LNPs highly appealing for therapeutic applications. LNPs are versatile carriers capable of delivering a wide range of substances, including pDNA, mRNA, siRNA, and proteins. This versatility is particularly valuable in CRISPR-based therapies, as LNPs can simultaneously deliver various CRISPR components within a single vehicle. These components may include mRNA or pDNA encoding the Cas nuclease, guide RNA, or even DNA donor templates used in homology-directed repair-based genome editing. Additionally, LNPs allow for easy modulation of particle size, surface properties, composition, and formulation to minimize toxicity and optimize tissue targeting. Such adaptability makes them an invaluable platform for advancing gene therapy.
Future of Gene Therapy
While the LNP platform holds great promise, it also faces several significant challenges. First, intravenous administration of current LNP formulations often results in infusion-related reactions. For example, the gene therapy drug patisiran is typically administered following premedication with antihistamines, acetaminophen, and dexamethasone to mitigate these reactions. Addressing this limitation is crucial for expanding LNP-based gene therapies to treat other diseases. Second, LNPs predominantly target the liver. After intravenous injection, 80-90% of LNPs accumulate in the liver, where hepatocytes absorb them via low-density lipoprotein (LDL) receptors. A key challenge lies in developing methods to bypass hepatic targeting and effectively deliver gene therapy payloads to non-liver tissues following systemic administration. Third, the large-scale production of functional lipids and LNPs remains a bottleneck. Currently, only a limited number of companies can manufacture these materials at scale. Notably, the only instance of large-scale LNP production in human history has been for COVID-19 vaccines.
Following the success of the Pfizer and Moderna COVID-19 vaccines, an influx of mRNA vaccines based on LNP technology is anticipated for other diseases, such as cancer. Additionally, new ionizable cationic lipids are needed for safer intravenous administration. The optimized design of LNPs is essential for achieving systemic delivery to non-liver tissues. From a broader perspective, both viral and non-viral vector platforms will continue to mature, each contributing to gene therapy in distinct ways. For example, DNA-based therapies, such as gene replacement or addition therapies, require DNA delivery to the cell nucleus. Consequently, adeno-associated virus (AAV) vectors are expected to remain dominant in such applications. Conversely, mRNA-based therapies, which only require cytoplasmic delivery, are more suitable for non-viral vectors like LNPs.
Moreover, LNPs are particularly adept at delivering complex substances, such as the various components of CRISPR-Cas genome editing therapies. A unique advantage of LNPs in these applications is their ability to enable transient expression of Cas nucleases, reducing off-target effects. In contrast, AAV-mediated delivery often results in prolonged Cas nuclease expression. As delivery technologies improve, the use of in vivo gene therapy is expected to expand, potentially replacing ex vivo approaches in some cases. If in vivo delivery can achieve sufficient precision, safety, and efficacy, the demand for ex vivo methods will naturally decline. Ultimately, both viral and non-viral platforms will secure their respective places in the future landscape of gene therapy.
** Recommended Products **
Catalog | Name | CAS | Price |
---|---|---|---|
BPG-3033 | 16:0 propargyl SM (d18:1-16:0) | 1196670-47-9 | Inquiry |
BPG-3036 | C6(6-azido) LacCer | 2260670-19-5 | Inquiry |
BPG-3042 | 18:0 azidoethyl PC | 2342574-09-6 | Inquiry |
BPG-3048 | 16:0(alkyne)-18:1 PC | 1633800-00-6 | Inquiry |
BPG-3072 | 18-NBD 18:1 Coenzyme A | 2410279-75-1 | Inquiry |
BPG-3109 | 12:0 Diether PC | 72593-72-7 | Inquiry |
BPG-3254 | Ent-25-hydroxycholesterol | 915159-32-9 | Inquiry |
BPG-3268 | BODIPY-Cholesterol | 878557-19-8 | Inquiry |
BPG-3281 | 27-Hydroxycholesterol | 20380-11-4 | Inquiry |
BPG-3287 | 25-Hydroxy-Cholesterol | 2140-46-7 | Inquiry |
BPG-3290 | 24(S)-Hydroxycholesterol | 474-73-7 | Inquiry |
BPG-3291 | 24(R)-hydroxycholesterol | 27460-26-0 | Inquiry |
BPG-3309 | 25, 27-dihydroxycholesterol | 55648-25-4 | Inquiry |
BPG-3310 | 7β,25-dihydroxycholesterol | 64907-21-7 | Inquiry |
BPG-3652 | BP Lipid 229 | 2089251-55-6 | Inquiry |
BPG-3705 | BP Lipid 112 | 2714482-37-6 | Inquiry |
Reference
- Zhao, Y. et al. Lipid nanoparticles for gene delivery. Adv Genet. 2014, 88: 13-36.
Why BOC Sciences?
-
Large Stock
More than 2000+ products in inventory
-
Global Delivery
Warehouses in multiple cities to ensure fast delivery
-
mg to kg
Multi specification for academic research and industrial production
-
24/7 Technical Support
Strict process parameter control to ensure product quality
Technical Support
- Aqueous Two-Phase System (ATPS) Technique
- Capillary Electrophoresis (CE) Technique
- Enzyme-linked immunosorbent assay (ELISA) Technique
- High performance liquid chromatography (HPLC) Technique
- Hydrophobic Interaction Chromatography (HIC) Technique
- PEGylated Protein Purification Techniques
- Radiolabeling Technique
- SDS-PAGE Technique
- Ultrafiltration Technique
Products
- Lipids
- PEG Derivatives by Structure
-
PEG Derivatives by Functional Group
- Acrylate/Acrylamide/Methacrylate PEG
- Aldehyde (Ald/CHO)PEG
- Alkyne PEG
- Amino PEG, PEG amine(-NH2)
- Azide PEG, Azido PEG(-N3)
- Biotin PEG
- Boc/Fmoc protected amine PEG
- Carboxylic Acid(-COOH) PEG
- Cholesterol PEG
- DBCO PEG
- DNP PEG
- DSPE PEG
- Epoxide glycidyl ether PEG
- FITC PEG
- Folate PEG
- Halide (chloride, bromide) PEG
- Hydrazide PEG
- Hydroxyl(-OH) PEG
- Maleimide(-MAL) PEG
- NHS ester PEG
- Nitrophenyl carbonate (NPC) PEG
- Norbornene PEG
- Olefin/Alkene/Vinyl PEG
- Orthopyridyl disulfide (OPSS) PEG
- Phosphate PEG
- Rhodamine PEG
- SCM PEG
- Silane PEG
- SPDP PEG
- Sulfonate (tosyl, mesyl, tresyl) PEG
- tert-Butyl protected carboxylate PEG
- Thiol(-SH) PEG
- Vinylsulfone PEG
- PEG Copolymers
-
PEG Raw Materials
- Small-molecule Polyethylene Glycol
- Polyethylene Glycol 1000
- Polyethylene Glycol 10000
- Polyethylene Glycol 1500
- Polyethylene Glycol 200
- Polyethylene Glycol 2000
- Polyethylene Glycol 20000
- Polyethylene Glycol 400
- Polyethylene Glycol 4000
- Polyethylene Glycol 600
- Polyethylene Glycol 6000
- Polyethylene Glycol 800
- Polyethylene Glycol 8000
Resources
-
Technical Information
- Aqueous Two-Phase System (ATPS) Technique
- Capillary Electrophoresis (CE) Technique
- Enzyme-linked immunosorbent assay (ELISA) Technique
- High performance liquid chromatography (HPLC) Technique
- How to Perform Polyethylene Glycol (PEG) Modification?
- Hydrophobic Interaction Chromatography (HIC) Technique
- Introduction of Polyethylene Glycol (PEG)
- Ion Exchange Chromatography (IEX) Technique
- PEG for Chemical Synthesis
- PEG for Cosmetic Application
- PEG for Drug Delivery
- PEG for Imaging Diagnosis
- PEG for Pharmaceutical Preparation
- PEG for Tissue Engineering
- PEG Purification Techniques of Plasmid DNA
- PEGylated Protein Purification Techniques
- Polyethylene Glycol (PEG) Modifier Selection Guide
- Radiolabeling Technique
- SDS-PAGE Technique
- Size Exclusion Chromatography (SEC) Technique
- Ultrafiltration Technique
-
Industry News
- Applications of PEG-DSPE: Drug Carriers and Drug Delivery
- Applications of Polyethylene Glycol (PEG) as Medical Devices
- Cholesterol: Definition, Structure, Synthesis, Types and Functions
- Classification of Lipid-Based Vaccine Adjuvants
- FDA approved PEGylated Products
- FDA-Approved Antibody-Drug Conjugates up to 2024
- How are Liposomes Different from Micelles?
- How Lipid Nanoparticles (LNPs) Deliver RNA Drugs?
- Hyaluronic Acid & PEGylated Hyaluronic Acid
- Ionizable Lipids for RNA Delivery
- Lipid Classification and Drug Delivery Systems
- Lipid Formulations: Key Absorption-Enhancing Technologies in New Drug Development
- Lipid-Drug Conjugates (LDCs) for Nanoparticle Drug Delivery
- Liposome in Drug Delivery
- Overview of Liposome Preparation Process
- PEG in Pharmaceutical Preparations (I): Solvents, Lubricants, Adhesives and More
- PEG in Pharmaceutical Preparations (II): Stabilizers, Plasticizers and Modification Materials
- PEG Linkers in Antibody Drug Conjugates and PROTACs
- PEG-DSPE Block Copolymers and Their Derivatives
- PEGylated Drugs: Definition, Structure, Classification and Benefits
- PEGylated RGD Peptides: A Promising Tool for Targeted Drug Delivery
- Pharmacokinetics and Bioanalysis of PEGylated Drugs
- Polyethylene Glycol (PEG) Modified Targeting Nanomaterials
- Preparation Method of PEG Hydrogel
- The PROTAC Technology in Drug Development
- Vaccines: Definition, History, Ingredients, Types and Mechanism of Action
- What are Lipid Excipients and Their Applications?
- What are Lipid Nanoparticles and Their Applications?
- What are Lipid-Drug Conjugates (LDCs)?
- What are Lipids?
- What are Monodispersed and Polydispersed PEGs?
- What are PEG Lipids?
- What are Phospholipids?
- What are Sterols? - Definition, Structure, Function, Examples and Uses
- What is Biotinylation and Biotinylated PEG?
- What is Click Chemistry?
- What is Hydrogel?
- What is Methoxy Polyethylene Glycol (mPEG)?
- What is Nanogels and Its Applications?
- What is the Formulation of Lipid Nanoparticles (LNPs)?
Our Feature
BOC Sciences supplies a unique variety of PEG derivatives and functional PEG polymers. Our products offer the most diverse collection of reactivity, ready-to-use functionality, and molecular weight options that you will not find anywhere else.
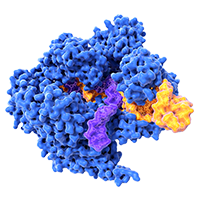
PEGylation of Peptides
and Proteins
Reduce the Immunogenicity of Peptide/Protein Drugs
Learn More
APPLICATIONS
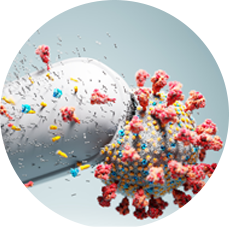
PEG linkers For Drug
Improved Circulation Half-Life
Learn More
Have Customer
Reviewed On Us?
Chat With Us