Ionizable Lipids for RNA Delivery
Broadly speaking, ribonucleic acid (RNA) therapy includes CRISPR-Cas9 systems mediated by antisense oligonucleotides (ASOs), small interfering RNA (siRNA), microRNA (miRNA), messenger RNA (mRNA), and single-guide RNA (sgRNA), which can manipulate any gene of interest through different modes of action. However, RNA therapeutics are sensitive to nucleases and cannot penetrate cells due to their large size and negative charge. Delivery of RNA to target cells via clinically translatable lipid nanoparticles (LNPs) offers tremendous opportunities to address a range of life-threatening diseases, including COVID-19.
Fig. 1. Ionizable lipids for RNA delivery (Nat Commun. 2021, 12(1): 7233).
LNPs are usually composed of four components-ionizable lipids, phospholipids, cholesterol, and PEGylated lipids, among which ionizable lipids play a major role in protecting RNA and promoting its cytoplasmic transport. Ionizable lipids are positively charged at acidic pH to condense RNA into LNPs, but are neutral at physiological pH to minimize toxicity. They can be protonated in acidic endosomes after cellular uptake and interact with anionic endosomal phospholipids to form conical ion pairs that are incompatible with the bilayer. These cationic-anionic lipid pairs drive the transition of the bilayer structure to the inverted hexagonal HII phase, thereby promoting membrane fusion/disruption, endosomal escape, and cargo release into the cytoplasm. Since 2008, ionizable lipids with diverse chemical properties have been created. A systematic classification of these lipids based on their structure could greatly benefit the field and facilitate the development of the next generation of ionizable lipids. Currently, there are five major ionizable lipid types widely used for RNA delivery.
Raw Materials for Lipid Nanoparticles
BOC Sciences is a leading supplier of high-quality lipid nanoparticle raw materials, offering a broad range of ionizable lipids, phospholipids, cholesterol and PEGylated lipids to support research and development in the area of drug delivery systems. With an unwavering commitment to quality, innovation and customer satisfaction, BOC Sciences has become a trusted partner to pharmaceutical companies, research institutions and academic laboratories worldwide. We are committed to supporting innovative research and advancing the advancement of lipid nanoparticle-based drug delivery technologies.
Classification of Ionizable Lipids
Unsaturated Ionizable Lipids
Tail saturation greatly affects the mobility and delivery efficiency of ionizable lipids. Increasing tail unsaturation from 0 to 2 cis double bonds is associated with an increased tendency of bilayer lipids to form a nonbilayer phase, resulting in membrane disruption and enhanced payload release. Therefore, during the optimization process of the ionizable lipid DLin-MC3-DMA (MC3), the linoleyl tail was selected as the structural basis. This ionizable lipid has potent hepatic gene silencing effects and is used in the first FDA-approved siRNA drug, Onpattro®, to treat hereditary transthyretin amyloidosis (hATTR). Importantly, the structure and formulation of MC3 lay the foundation for further development of LNPs.
Unsaturated ionizable lipids have also been shown to enhance mRNA delivery. Anderson and colleagues demonstrated that a linoleic acid-derived ionizable lipid (OF-02) achieved the highest hepatic mRNA delivery and protein expression among similar lipids. Later, they demonstrated that an alkyne-ionizable lipid (A6) can greatly increase membrane fusion and facilitate albumin-mediated mRNA delivery when incorporated into a benchmark LNP such as MC3.
Multi-tail Ionizable Lipids
Multi-tailed ionizable lipids differ structurally from two-tailed ionizable lipids in having three or more tails. Due to the increased cross-section of the tail region, this ionizable lipid is expected to produce a more tapered structure with enhanced endosomal destruction capabilities. Multi-tailed ionized lipids can be easily synthesized via combinatorial chemistry and subjected to high-throughput screening.
Although originally developed for siRNA delivery, multi-tailed ionizable lipids can be repurposed for efficient mRNA delivery through experimental design to optimize LNP composition and significantly reduce workload. For example, an optimized formulation of C12-200 resulted in a 7-fold increase in mRNA expression compared to the standard formulation. Later, this formulation was widely adopted as multi-tailed ionized lipids for various mRNA delivery purposes, such as chimeric antigen receptor T-cell engineering and prenatal protein replacement therapy.
Ionizable Polymer Lipids
Substitution of free amines on cationic polymers with alkyl tails provides ionizable polymeric lipids that can enhance particle formation through hydrophobic aggregation. From 500 structurally diverse synthetic polymer-lipid hybrids, Anderson et al. determined that a C15 epoxide-modified low molecular weight polyethylenimine (7C1) had the most efficient non-hepatic siRNA delivery. 7C1 preferentially transfects endothelial cells over liver cells and lung epithelial cells in multiple organs, with the highest degree of endothelial gene silencing in the lung. In non-human primates, 7C1 achieved ~80% knockout of lung endothelial genes without significant toxicity, suggesting its potential to treat dysfunctional endothelial-related diseases. Recently, its re-optimized formula has been further proven to achieve effective gene silencing in bone marrow endothelial cells, which can further regulate hematopoietic cell activity.
Biodegradable Ionizable Lipids
To reduce accumulation and potential side effects, ionizable lipids should be readily degraded into nontoxic metabolites upon successful intracellular cargo delivery, which is particularly important for RNA therapeutics that require repeated administration. A common strategy to introduce biodegradability into ionizable lipids is through the inclusion of ester linkages that are stable at physiological pH but are enzymatically hydrolyzed within tissues and cells. For example, due to the slow degradability of the dilinoleyl tails in MC3, biodegradable substituents were created by replacing one double bond in each tail with a primary ester (L319). L319 not only maintained in vivo potency but also demonstrated rapid elimination and improved tolerability. Notably, the position and steric effects of the ester group can greatly affect the clearance and potency of ionizable lipids.
Branched-tail Ionizable Lipids
Like tail length and saturation, tail branching can greatly affect the performance of ionizable lipids. Ionizable lipids with a methacrylate tail (1C branch near the head) generally exhibit lower efficacy compared to acrylate-based lipids. Interestingly, an ionizable lipid containing isodecyl acrylate (Oi10, terminal 1C branch) significantly increased liver mRNA expression (>10-fold) compared to isomers with linear tails, despite their similar biological distribution. This increase in potency can be explained by enhanced endosomal escape due to stronger protonation of the ionizable lipids spaced at endosomal pH and an increase in the cross-section of the lipid tail, allowing the adoption of a more tapered structure. Lead ionizable lipids (306Oi10) effectively co-deliver a variety of RNA constructs to the liver and transfect >80% of hepatocytes, Kupffer cells, and endothelial cells, demonstrating the potential of integrating different treatments (such as gene silencing, expression, and editing) to treat liver dysfunction.
Our Lipid Support Services
BOC Sciences' lipid support services cover a broad range of capabilities to meet the diverse needs of lipid researchers and industry. From custom lipid synthesis to lipid analysis, modification, packaging and formulation, we provide comprehensive solutions to support lipid research and development efforts. By partnering with BOC Sciences, researchers can gain access to the expertise, resources and technology they need to deepen their understanding of lipid biology and develop novel lipid products with potential applications in pharmaceutical research, biotechnology and more.
Clinical Development of Ionizable Lipids
Since Onpattro® was approved in 2018, scientists have screened multiple ionizable lipid and LNP formulations. The success of MC3 has reignited enthusiasm for RNA delivery and greatly accelerated the clinical development of other LNP-based RNA therapeutics, especially mRNA vaccines. Specifically, the COVID-19 pandemic has killed millions of people. However, in less than a year, two LNP-based mRNA vaccines (mRNA-1273 and BNT162b2) were developed at an unprecedented pace and received historic emergency use approval after demonstrating over 94% protective efficacy. Interestingly, Moderna's SM-102 and BioNTech's Acuitas ALC-0315 share some common features, including tertiary amines, branched tails, and ester linkers. Additionally, they all have extended aliphatic branches that make them look like multi-tailed structures. Other COVID-19 mRNA vaccines offered by LNP are currently in clinical development. Although the structures of these ionizable lipids have not yet been published, possible structures are shown based on existing patents and literature (Fig. 2).
Fig. 2. Ionizable lipids in clinical development (Nat Commun. 2021, 12(1): 7233).
When reviewing the approved structures of MC3, SM-102, and ALC-0315 as well as the patented structures of Acuitas et al., ester-based biodegradable structures appeared in each of the ionizable lipids; the next most common feature was poly/branched tailed and unsaturated structure. This suggests that biodegradability is a key feature for the clinical translation of ionizable lipids. Furthermore, an increased number of branches or tails is a common feature of newly developed ionizable lipids. However, these poly/branched tailed ionizable lipids typically have only one tertiary amine. Interestingly, while unsaturated structure is important for ionizable lipids with long tails, it appears to be less important for ionizable lipids with multiple short tails.
Reference
- Mitchell, M.J. et al. An ionizable lipid toolbox for RNA delivery. Nat Commun. 2021, 12(1): 7233.
Why BOC Sciences?
-
Large Stock
More than 2000+ products in inventory
-
Global Delivery
Warehouses in multiple cities to ensure fast delivery
-
mg to kg
Multi specification for academic research and industrial production
-
24/7 Technical Support
Strict process parameter control to ensure product quality
Technical Support
- Aqueous Two-Phase System (ATPS) Technique
- Capillary Electrophoresis (CE) Technique
- Enzyme-linked immunosorbent assay (ELISA) Technique
- High performance liquid chromatography (HPLC) Technique
- Hydrophobic Interaction Chromatography (HIC) Technique
- PEGylated Protein Purification Techniques
- Radiolabeling Technique
- SDS-PAGE Technique
- Ultrafiltration Technique
Products
- Lipids
- PEG Derivatives by Structure
-
PEG Derivatives by Functional Group
- Acrylate/Acrylamide/Methacrylate PEG
- Aldehyde (Ald/CHO)PEG
- Alkyne PEG
- Amino PEG, PEG amine(-NH2)
- Azide PEG, Azido PEG(-N3)
- Biotin PEG
- Boc/Fmoc protected amine PEG
- Carboxylic Acid(-COOH) PEG
- Cholesterol PEG
- DBCO PEG
- DNP PEG
- DSPE PEG
- Epoxide glycidyl ether PEG
- FITC PEG
- Folate PEG
- Halide (chloride, bromide) PEG
- Hydrazide PEG
- Hydroxyl(-OH) PEG
- Maleimide(-MAL) PEG
- NHS ester PEG
- Nitrophenyl carbonate (NPC) PEG
- Norbornene PEG
- Olefin/Alkene/Vinyl PEG
- Orthopyridyl disulfide (OPSS) PEG
- Phosphate PEG
- Rhodamine PEG
- SCM PEG
- Silane PEG
- SPDP PEG
- Sulfonate (tosyl, mesyl, tresyl) PEG
- tert-Butyl protected carboxylate PEG
- Thiol(-SH) PEG
- Vinylsulfone PEG
- PEG Copolymers
-
PEG Raw Materials
- Small-molecule Polyethylene Glycol
- Polyethylene Glycol 1000
- Polyethylene Glycol 10000
- Polyethylene Glycol 1500
- Polyethylene Glycol 200
- Polyethylene Glycol 2000
- Polyethylene Glycol 20000
- Polyethylene Glycol 400
- Polyethylene Glycol 4000
- Polyethylene Glycol 600
- Polyethylene Glycol 6000
- Polyethylene Glycol 800
- Polyethylene Glycol 8000
Resources
-
Technical Information
- Aqueous Two-Phase System (ATPS) Technique
- Capillary Electrophoresis (CE) Technique
- Enzyme-linked immunosorbent assay (ELISA) Technique
- High performance liquid chromatography (HPLC) Technique
- How to Perform Polyethylene Glycol (PEG) Modification?
- Hydrophobic Interaction Chromatography (HIC) Technique
- Introduction of Polyethylene Glycol (PEG)
- Ion Exchange Chromatography (IEX) Technique
- PEG for Chemical Synthesis
- PEG for Cosmetic Application
- PEG for Drug Delivery
- PEG for Imaging Diagnosis
- PEG for Pharmaceutical Preparation
- PEG for Tissue Engineering
- PEG Purification Techniques of Plasmid DNA
- PEGylated Protein Purification Techniques
- Polyethylene Glycol (PEG) Modifier Selection Guide
- Radiolabeling Technique
- SDS-PAGE Technique
- Size Exclusion Chromatography (SEC) Technique
- Ultrafiltration Technique
-
Industry News
- Applications of PEG-DSPE: Drug Carriers and Drug Delivery
- Applications of Polyethylene Glycol (PEG) as Medical Devices
- Cholesterol: Definition, Structure, Synthesis, Types and Functions
- Classification of Lipid-Based Vaccine Adjuvants
- FDA approved PEGylated Products
- FDA-Approved Antibody-Drug Conjugates up to 2024
- How are Liposomes Different from Micelles?
- How Lipid Nanoparticles (LNPs) Deliver RNA Drugs?
- Hyaluronic Acid & PEGylated Hyaluronic Acid
- Ionizable Lipids for RNA Delivery
- Lipid Classification and Drug Delivery Systems
- Lipid Formulations: Key Absorption-Enhancing Technologies in New Drug Development
- Lipid-Drug Conjugates (LDCs) for Nanoparticle Drug Delivery
- Liposome in Drug Delivery
- Overview of Liposome Preparation Process
- PEG in Pharmaceutical Preparations (I): Solvents, Lubricants, Adhesives and More
- PEG in Pharmaceutical Preparations (II): Stabilizers, Plasticizers and Modification Materials
- PEG Linkers in Antibody Drug Conjugates and PROTACs
- PEG-DSPE Block Copolymers and Their Derivatives
- PEGylated Drugs: Definition, Structure, Classification and Benefits
- PEGylated RGD Peptides: A Promising Tool for Targeted Drug Delivery
- Pharmacokinetics and Bioanalysis of PEGylated Drugs
- Polyethylene Glycol (PEG) Modified Targeting Nanomaterials
- Preparation Method of PEG Hydrogel
- The PROTAC Technology in Drug Development
- Vaccines: Definition, History, Ingredients, Types and Mechanism of Action
- What are Lipid Excipients and Their Applications?
- What are Lipid Nanoparticles and Their Applications?
- What are Lipid-Drug Conjugates (LDCs)?
- What are Lipids?
- What are Monodispersed and Polydispersed PEGs?
- What are PEG Lipids?
- What are Phospholipids?
- What are Sterols? - Definition, Structure, Function, Examples and Uses
- What is Biotinylation and Biotinylated PEG?
- What is Click Chemistry?
- What is Hydrogel?
- What is Methoxy Polyethylene Glycol (mPEG)?
- What is Nanogels and Its Applications?
- What is the Formulation of Lipid Nanoparticles (LNPs)?
Our Feature
BOC Sciences supplies a unique variety of PEG derivatives and functional PEG polymers. Our products offer the most diverse collection of reactivity, ready-to-use functionality, and molecular weight options that you will not find anywhere else.
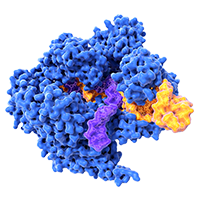
PEGylation of Peptides
and Proteins
Reduce the Immunogenicity of Peptide/Protein Drugs
Learn More
APPLICATIONS
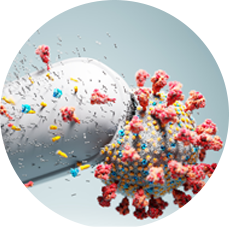
PEG linkers For Drug
Improved Circulation Half-Life
Learn More
Have Customer
Reviewed On Us?
Chat With Us