How Lipid Nanoparticles (LNPs) Deliver RNA Drugs?
Lipid nanoparticles (LNPs) are lipid vesicles with a uniform lipid core that are widely used for the delivery of small molecule and nucleic acid drugs and have recently attracted much attention due to their great success as a COVID-19 mRNA vaccine delivery platform. The applications of transient protein expression induced by mRNA extend far beyond infectious disease vaccines. It also has huge potential application value in cancer vaccines, protein replacement therapies, and gene editing components for rare genetic diseases. However, naked mRNA itself is extremely unstable and easily degraded rapidly by nucleases and autohydrolysis. Encapsulation of LNP protects mRNA from extracellular RNases and assists intracellular delivery of mRNA.
How are LNPs Manufactured?
The preparation of LNPs depends on the self-assembly ability, that is, the lipid components spontaneously organize into nanostructured entities through intermolecular interactions. First, there is an electrostatic bond between negatively charged nucleic acids and positively charged lipids. Then, LNPs are assembled through hydrophobic interactions and van der Waals interactions between lipid components. Characterization of the early stages of self-assembly and study of the associated effects on the final properties of LNPs remains challenging due to the diversity of lipid chemistries, the uniqueness of nucleic acids, and the temporal nature of the mixing of the two. The LNP preparation protocol will affect the self-assembly product in at least two aspects: the uniformity of the LNP and the nucleic acid loading efficiency.
There are many methods for LNP preparation, including liposome extrusion, film hydration, nanoprecipitation, and microfluidics. Among them, the most commonly used method is the rapid mixing method of aqueous phase and lipid components. The microfluidic method has good reproducibility and has become the method of choice for preclinical research. Recently, technological advances in microfluidic devices have made this method easier to implement. While microfluidic mixing often facilitates encapsulation of hydrophilic moieties into hydrophobic lipid cores, there are no strict requirements for the introduction of nucleic acids. In conclusion, the nature of the mixing method may affect the loading efficiency and internal organizational form of LNPs, as the structure of the nanoparticles appears to be determined by the kinetic factors of self-assembly.
Our LNP Development Services
BOC Sciences can provide custom manufacturing services for LNP production. We have state-of-the-art facilities and an experienced team of scientists to design and optimize lipid formulations to meet our customers' specific requirements. BOC Sciences' manufacturing capabilities include lipid synthesis, formulation development, and scale-up production of LNPs for preclinical and clinical studies.
What is the Formulation of LNPs?
Currently, the lipid components of classic LNPs usually include four types: ionizable lipids (or cationic lipids), auxiliary lipids, cholesterol, and pegylated lipids (PEG-lipids).
- Cationic lipids (CLs) and ionizable lipids (ILs): Cationic lipids (CLs) and ionizable lipids (ILs) can initiate the first step of self-assembly through electrostatic interactions.
- Cholesterol: Cholesterol is a naturally abundant component of cell membranes and is often used as a structural lipid in LNP formulations. Cholesterol accounts for about 20-50% in LNP formula.
- Phospholipids: Phospholipids help encapsulate nucleic acids and stabilize LNPs. Compared with other components, there are relatively few studies on them, and they usually account for 10%-20% of the total lipids in the formula.
- PEG-modified lipids: PEG-modified lipids (PEG-Lipids) are an important component of LNPs that regulate half-life and cellular uptake.
Our Lipids for Lipid Nanoparticles
BOC Sciences is a leading supplier of lipid products, offering comprehensive manufacturing capabilities for LNP production. We have a broad range of lipid products including phospholipids, cholesterol and other lipid components necessary for LNP formulations. BOC Sciences' lipid products are of high quality and purity suitable for use in pharmaceutical and biotechnology applications.
Catalog | Product Name | CAS Number | Category |
---|---|---|---|
BPG-3287 | 25-Hydroxy-Cholesterol | 2140-46-7 | Glycosylated Sterols |
BPG-3312 | 7Keto-25-hydroxy cholesterol | 64907-23-9 | Oxysterols |
BPG-3294 | 20(S)-Hydroxycholesterol | 516-72-3 | Natural Sterols |
BPG-3299 | 7β,27-dihydroxy Cholesterol | 240129-43-5 | Oxysterols |
BPG-3271 | 3-hexanoyl-NBD Cholesterol | 201731-19-3 | Phospholipids |
BPG-3270 | 3-dodecanoyl-NBD Cholesterol | 1246303-05-8 | Phospholipids |
BPG-3272 | 16:0 TopFluor® cholesterol | 2260795-57-9 | Phospholipids |
BPG-3273 | 18:2 TopFluor® cholesterol | 2260795-60-4 | Phospholipids |
BPG-3269 | 25-(C4 TopFluor®) 25-OH cholesterol | 2260795-67-1 | Phospholipids |
BPG-2994 | DOPE PEG(2000)-N-Cy5 | 2389048-58-0 | mPEG Phospholipids |
What are the Properties of Lipid Nanoparticles?
- The average particle size and particle size distribution of LNPs are important initial determinants of LNP quality and suitability for various applications. These features are often characterized by dynamic light scattering (DLS). Generally, the optimal particle size of LNP is 20-200 nm, as this size makes the LNP strong enough to withstand fluid flow (such as blood and lymph) while allowing the LNP to pass through the interstitium. LNP size is usually tuned by changing the amount of PEG-lipid or mixing parameters such as flow rate and volume ratio. LNP size may affect LNP internalization, biodistribution, degradation, and clearance, and different applications may require different particle sizes. For example, in mouse models, 45nm-siRNA-LNP delivered the most efficiently when administered subcutaneously, while 80nm-siRNA-LNP was most effective when administered intravenously. However, comparison of different mRNA-LNP particle sizes in rodents and non-human primates showed that non-human primates are not that sensitive to particle size when LNP is injected intramuscularly.
- The surface charge of LNPs is responsible for interactions with cell membranes and the biological environment. Because the cell membrane is negatively charged, the negatively charged LNP on the surface will repel the cell membrane and will not be absorbed by the cells. On the other hand, positively charged LNPs may directly damage cell membranes, causing cytotoxicity. This is why ionizable lipids are crucial in LNP design. Initially, LNPs containing ionizable lipids are electrically neutral, avoiding any unwanted electrostatic interactions, but acquire a positive charge at the acidic pH of the endosome. The surface charge of LNPs is usually evaluated by zeta potential measurements. This technique is commonly used to evaluate colloid aggregation. Although there is no strict classification, the surface charge is considered weak if the zeta potential is between -20 and +20 mV.
- A common method to adjust the total surface charge of LNPs is to adjust the N/P ratio, that is, the ratio of ionizable lipids (N, representing cationic amines) to nucleic acids (P, representing anionic phosphates). Carrasco et al. reported that in LNPs containing ionizable lipid KC2, increasing the N/P ratio can increase the surface charge and encapsulation efficiency. Furthermore, the introduction of permanently charged lipids into LNPs may alter the preferential uptake tendencies of organs without increasing surface charge. Cheng et al. implemented lipid charge-based selective organ targeting (SORT) in mice: adding positively charged lipids to the LNP formulation preferentially targets lung tissue for transfection, while negatively charged lipids preferentially target transfection directly to the spleen.
- Lipid accumulation may affect many parameters, from membrane hydration and deformability to cellular uptake and cargo release. Briefly, each lipid can be described by a packing parameter that depends on the volume occupied by a polar "head" and a nonpolar "tail." Structurally homogeneous lipids form cylindrical structures and lamellar phases, while heterogeneous lipids form hexagonal, cubic, and micellar phases. The inverse hexagonal phase (HII) appears to promote lipid membrane fusion most significantly. Currently, in the field of RNA-LNP delivery, controllable preparation of non-lamellar phases, such as cubic phases, is relatively rare. However, LNPs may undergo structural changes when environmental triggers are present.
- Depending on the lipid phase and overall polarity, lipid membranes may entrain water, thereby altering membrane fluidity or deformability, thereby affecting lipid membrane fusion. Membrane hydration may also affect potential responsiveness to pH, a key environmental trigger commonly used to initiate the release of nucleic acids. When LNP enters the intracellular space, it becomes trapped in endosomes, which gradually acidify as they mature into lysosomes. Therefore, higher water content in lipid membranes may affect acidification kinetics and contribute to rapid membrane destabilization. Koitabashi et al. used Laurdan analysis to detect the response of lipid membrane destabilization in siRNA-LNP to pH and found that membrane hydration was positively correlated with gene silencing efficiency.
- The inherent aqueous environment of LNP also poses a threat to its long-term stability. Under normal environmental conditions, pure nucleic acids can rapidly deteriorate through exogenous RNase degradation or autohydrolysis. Although LNP can protect nucleic acids from enzymatic degradation, due to thermodynamic factors (such as minimizing phase separation), LNPs tend to aggregate, causing nucleic acids to be lost upon nanoparticle fusion, ultimately affecting transfection efficiency. Low-temperature storage and freeze-drying may preserve RNA but damage LNPs due to the formation of ice crystals, a problem that appears to be alleviated by adding cryoprotectants such as sucrose. Interestingly, LNPs may change their preferential uptake into organs depending on storage conditions, possibly as a result of their reorganization.
LNP is a highly customizable nucleic acid delivery vector that shows great potential in mRNA vaccine delivery. In addition, the potential value of LNP in rare disease and cancer treatment cannot be ignored. mRNA therapies can help produce therapeutic proteins to restore function to damaged tissues or organs. Extensive research has been conducted globally to design and refine the individual components of LNPs for efficient and safe delivery of target nucleic acids. If you are interested in our lipid products or lipid services, please contact us for more information.
Why BOC Sciences?
-
Large Stock
More than 2000+ products in inventory
-
Global Delivery
Warehouses in multiple cities to ensure fast delivery
-
mg to kg
Multi specification for academic research and industrial production
-
24/7 Technical Support
Strict process parameter control to ensure product quality
Technical Support
- Aqueous Two-Phase System (ATPS) Technique
- Capillary Electrophoresis (CE) Technique
- Enzyme-linked immunosorbent assay (ELISA) Technique
- High performance liquid chromatography (HPLC) Technique
- Hydrophobic Interaction Chromatography (HIC) Technique
- PEGylated Protein Purification Techniques
- Radiolabeling Technique
- SDS-PAGE Technique
- Ultrafiltration Technique
Products
- Lipids
- PEG Derivatives by Structure
-
PEG Derivatives by Functional Group
- Acrylate/Acrylamide/Methacrylate PEG
- Aldehyde (Ald/CHO)PEG
- Alkyne PEG
- Amino PEG, PEG amine(-NH2)
- Azide PEG, Azido PEG(-N3)
- Biotin PEG
- Boc/Fmoc protected amine PEG
- Carboxylic Acid(-COOH) PEG
- Cholesterol PEG
- DBCO PEG
- DNP PEG
- DSPE PEG
- Epoxide glycidyl ether PEG
- FITC PEG
- Folate PEG
- Halide (chloride, bromide) PEG
- Hydrazide PEG
- Hydroxyl(-OH) PEG
- Maleimide(-MAL) PEG
- NHS ester PEG
- Nitrophenyl carbonate (NPC) PEG
- Norbornene PEG
- Olefin/Alkene/Vinyl PEG
- Orthopyridyl disulfide (OPSS) PEG
- Phosphate PEG
- Rhodamine PEG
- SCM PEG
- Silane PEG
- SPDP PEG
- Sulfonate (tosyl, mesyl, tresyl) PEG
- tert-Butyl protected carboxylate PEG
- Thiol(-SH) PEG
- Vinylsulfone PEG
- PEG Copolymers
-
PEG Raw Materials
- Small-molecule Polyethylene Glycol
- Polyethylene Glycol 1000
- Polyethylene Glycol 10000
- Polyethylene Glycol 1500
- Polyethylene Glycol 200
- Polyethylene Glycol 2000
- Polyethylene Glycol 20000
- Polyethylene Glycol 400
- Polyethylene Glycol 4000
- Polyethylene Glycol 600
- Polyethylene Glycol 6000
- Polyethylene Glycol 800
- Polyethylene Glycol 8000
Resources
-
Technical Information
- Aqueous Two-Phase System (ATPS) Technique
- Capillary Electrophoresis (CE) Technique
- Enzyme-linked immunosorbent assay (ELISA) Technique
- High performance liquid chromatography (HPLC) Technique
- How to Perform Polyethylene Glycol (PEG) Modification?
- Hydrophobic Interaction Chromatography (HIC) Technique
- Introduction of Polyethylene Glycol (PEG)
- Ion Exchange Chromatography (IEX) Technique
- PEG for Chemical Synthesis
- PEG for Cosmetic Application
- PEG for Drug Delivery
- PEG for Imaging Diagnosis
- PEG for Pharmaceutical Preparation
- PEG for Tissue Engineering
- PEG Purification Techniques of Plasmid DNA
- PEGylated Protein Purification Techniques
- Polyethylene Glycol (PEG) Modifier Selection Guide
- Radiolabeling Technique
- SDS-PAGE Technique
- Size Exclusion Chromatography (SEC) Technique
- Ultrafiltration Technique
-
Industry News
- Applications of PEG-DSPE: Drug Carriers and Drug Delivery
- Applications of Polyethylene Glycol (PEG) as Medical Devices
- Cholesterol: Definition, Structure, Synthesis, Types and Functions
- Classification of Lipid-Based Vaccine Adjuvants
- FDA approved PEGylated Products
- FDA-Approved Antibody-Drug Conjugates up to 2024
- How are Liposomes Different from Micelles?
- How Lipid Nanoparticles (LNPs) Deliver RNA Drugs?
- Hyaluronic Acid & PEGylated Hyaluronic Acid
- Ionizable Lipids for RNA Delivery
- Lipid Classification and Drug Delivery Systems
- Lipid Formulations: Key Absorption-Enhancing Technologies in New Drug Development
- Lipid-Drug Conjugates (LDCs) for Nanoparticle Drug Delivery
- Liposome in Drug Delivery
- Overview of Liposome Preparation Process
- PEG in Pharmaceutical Preparations (I): Solvents, Lubricants, Adhesives and More
- PEG in Pharmaceutical Preparations (II): Stabilizers, Plasticizers and Modification Materials
- PEG Linkers in Antibody Drug Conjugates and PROTACs
- PEG-DSPE Block Copolymers and Their Derivatives
- PEGylated Drugs: Definition, Structure, Classification and Benefits
- PEGylated RGD Peptides: A Promising Tool for Targeted Drug Delivery
- Pharmacokinetics and Bioanalysis of PEGylated Drugs
- Polyethylene Glycol (PEG) Modified Targeting Nanomaterials
- Preparation Method of PEG Hydrogel
- The PROTAC Technology in Drug Development
- Vaccines: Definition, History, Ingredients, Types and Mechanism of Action
- What are Lipid Excipients and Their Applications?
- What are Lipid Nanoparticles and Their Applications?
- What are Lipid-Drug Conjugates (LDCs)?
- What are Lipids?
- What are Monodispersed and Polydispersed PEGs?
- What are PEG Lipids?
- What are Phospholipids?
- What are Sterols? - Definition, Structure, Function, Examples and Uses
- What is Biotinylation and Biotinylated PEG?
- What is Click Chemistry?
- What is Hydrogel?
- What is Methoxy Polyethylene Glycol (mPEG)?
- What is Nanogels and Its Applications?
- What is the Formulation of Lipid Nanoparticles (LNPs)?
Our Feature
BOC Sciences supplies a unique variety of PEG derivatives and functional PEG polymers. Our products offer the most diverse collection of reactivity, ready-to-use functionality, and molecular weight options that you will not find anywhere else.
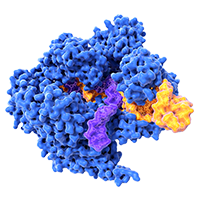
PEGylation of Peptides
and Proteins
Reduce the Immunogenicity of Peptide/Protein Drugs
Learn More
APPLICATIONS
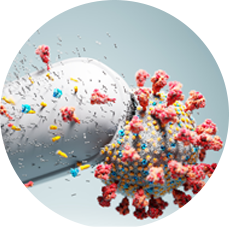
PEG linkers For Drug
Improved Circulation Half-Life
Learn More
Have Customer
Reviewed On Us?
Chat With Us