Drug Delivery: Definition, Advantages, Methods, Techniques, and Routes
Drug delivery is a key technology in the biopharmaceutical field, and its role in new drug development is becoming increasingly prominent. On one hand, emerging fields such as nucleic acid drugs, cell and gene therapies are flourishing, providing new solutions for many difficult-to-treat diseases. However, to implement these innovative therapies, it is essential to accurately and efficiently deliver therapeutic drugs to target cells or tissues in order to fully exert their therapeutic effects. Therefore, support from drug delivery technology is particularly important. On the other hand, the continuous development of drug delivery technology has strongly promoted new drug development. New delivery carriers, mechanisms, and intelligent delivery systems are constantly emerging, which not only improve the efficacy of drugs and reduce side effects but also provide more possibilities for new drug development. Drug delivery not only concerns the efficacy and safety of drugs but also directly affects whether a new drug can successfully reach the market.
Drug Delivery System
A drug delivery system refers to a technology that comprehensively regulates the distribution of drugs in the body in terms of space, time, and dosage. By enhancing the delivery of therapeutic drugs to their target sites and minimizing off-target accumulation, it improves patient health. Drugs must undergo a highly complex transport process in the body to exert their effects, and this transport is often influenced by various factors, which can reduce the drug's efficacy. Specifically, factors that affect drug transport include:
Fig. 1. Drug delivery systems (Curr Neuropharmacol. 2016, 14(4): 376-91).
- Physicochemical Properties of the Drug: The solubility, stability, polarity, and molecular weight of a drug directly determine its transport efficiency and distribution in the body. For example, low solubility may lead to poor absorption in the body, while poor stability could cause the drug to lose activity during transport.
- Characteristics of Biological Membranes: Biological membranes are the main barrier to drug transport, and their permeability, surface area, and thickness significantly impact the rate and amount of drug transport.
- Metabolism and Excretion of the Drug in the Body: After undergoing a series of metabolic processes in the body, the structure and activity of the drug may change, which in turn affects its transport and efficacy. Additionally, the rate of excretion impacts the drug's distribution in the body. A fast excretion rate could result in a short duration of action, making it difficult to achieve sustained therapeutic effects.
- Individual Biological Variations: Different individuals have variations in physiological structure, metabolic rate, and the distribution of drug receptors, which leads to differences in drug transport and efficacy across individuals.
Drug Delivery Advantages
Drug delivery enhances the delivery of drugs to target sites while minimizing off-target accumulation, thereby reducing toxicity and maximizing therapeutic effects. Specifically, the functions of drug delivery can be categorized into four key aspects:
- Controlled Drug Release: Controlled release systems allow drugs to be released slowly and continuously within the body, maintaining an effective therapeutic concentration window. This not only reduces the frequency of administration, improving patient adherence, but also prevents drastic fluctuations in drug concentrations, thus lowering the risk of side effects. For example, in the chronic disease field, certain sustained-release systems can provide drug release over periods of up to six months, significantly improving treatment outcomes and the patient's quality of life.
- Drug Targeting: By delivering drugs directly to the disease site, drug targeting reduces the impact on surrounding healthy tissues, improving treatment precision. This is especially beneficial for cancer therapies, where targeting cancer cells can reduce damage to normal cells.
- Enhanced Drug Stability: Many drugs exhibit poor stability in the body and are susceptible to enzymatic degradation or changes in pH and other environmental factors. Drug delivery systems can protect drug molecules through physical or chemical methods, improving their stability and prolonging their therapeutic action.
- Improved Drug Absorption: Some drugs, due to their physicochemical properties, are difficult for the body to absorb, such as macromolecular drugs and poorly soluble compounds. Drug delivery methods can enhance absorption by altering the drug's form (e.g., making nanoparticles) or using specialized carriers, particularly in overcoming biological barriers.
Drug Delivery Methods
From a research and development perspective, current drug delivery methods can be broadly divided into three categories: drug modification, microenvironment regulation, and drug delivery systems and devices.
Drug Modification
Drug modification refers to the chemical or biological transformation of drug molecules. By regulating interactions between drugs and molecules, cells, and tissues in the body, as well as between drugs and their targets, drug modification optimizes pharmacokinetic properties and therapeutic efficacy. It is applicable to improving the delivery of all categories of drugs, including small molecules, peptides, proteins, nucleic acids, and cells. Common drug modification methods include: first, covalent modification, where different molecular fragments are linked via covalent bonds to enhance drug properties; for example, attaching hydrophilic groups (such as polyethylene glycol) to hydrophobic drugs can increase their solubility in water. Second, conjugation modification, where drugs are conjugated with targeting molecules (such as antibodies, peptides, or aptamers) to enable specific recognition and binding to target cells or tissues, increasing drug concentration at the target site while reducing off-target distribution. Third, prodrug modification, where active drug molecules are chemically bonded to an inactive carrier, which releases the active drug through specific enzymatic or hydrolytic processes to achieve controlled release and targeted delivery. Fourth, glycosylation modification, where carbohydrate molecules are attached to drugs to improve their solubility, stability, and immunogenicity, as well as enhance interactions with cell surfaces, thereby improving cellular penetration.
Microenvironment Regulation
Microenvironment regulation works by adjusting the local environment of the drug within the body. On one hand, it can alter the conditions for drug absorption, such as modifying the pH of the gastrointestinal tract to enhance drug solubility. This approach is achieved using various excipients and additives. For example, Pfizer's COVID-19 drug Paxlovid includes a metabolic inhibitor to reduce liver metabolism and increase the bioavailability of the active drug. On the other hand, microenvironment regulation can modify factors that hinder drug action, such as using modified pH regulators to suppress protein degradation and improve the stability of proteins and peptides in physiological fluids.
Drug Delivery Systems and Devices
Drug delivery systems and devices facilitate controlled release by creating barriers between the drug and the body's environment. Based on the route of administration, these systems and devices can be categorized into oral, transdermal, implantable, inhalable, and injectable types. The main principle is to use natural materials such as lipids, proteins, and viruses, or synthetic materials like polymers, inorganic salts, and silica gels as carriers to encapsulate drugs. These systems use mechanisms like dissolution, diffusion, permeation, ion exchange, and degradation to control drug release. Drug delivery carriers are a frontier in current delivery systems, with natural carriers (such as extracellular vesicles like exosomes, microvesicles, and viruses, or cells like red blood cells and immune cells) and artificial carriers (such as liposomes, polymeric carriers, micelles, and other nanoparticles) being the main types.
Drug Delivery Techniques
The frontier of drug delivery technologies is characterized by diversity and innovation, with multiple techniques advancing in parallel, driving the evolution of drug delivery systems. Currently, the most advanced drug delivery methods include liposomes, microspheres, nanoparticles, and 3D printed drug formulations.
Hydrogel Drug Delivery
Hydrogels are polymer systems that are formed through simple reactions of one or more monomers, creating a three-dimensional network structure containing a large amount of water. As a polymer material, hydrogels swell upon contact with water and maintain a high water content within their structure, offering excellent biocompatibility and biodegradability. Their unique hydrophilic properties make them ideal for drug delivery, particularly for localized or targeted drug delivery. The mechanism of hydrogel drug delivery systems typically relies on physical or chemical interactions, where the drug is encapsulated within the network structure of the hydrogel. The rate of drug release is influenced by factors such as the hydrogel's crosslinking density, pore structure, and swelling ability. Hydrogels can also be engineered to respond to external stimuli, such as pH, temperature, or electric fields, enabling controlled release. This system is widely used in fields like anticancer drugs, protein drugs, vaccines, and gene therapy, offering advantages such as improved drug stability, extended therapeutic time, and reduced side effects.
Liposome Drug Delivery
Liposomes are closed vesicle structures formed by the self-assembly of amphiphilic molecules like phospholipids and cholesterol in water, capable of encapsulating both water-soluble and lipophilic drugs. This technology has several advantages: 1) It resembles the cell membrane, offering high biocompatibility and biodegradability, thus improving stability and drug dosage. 2) Liposomes can be chemically modified on their surface to enhance targeting, extend retention time at the disease site, and achieve precise treatment. 3) By altering surface charge, liposomes can be used to deliver nucleic acid drugs. Several liposome products are already available on the market, including doxorubicin, paclitaxel, and irinotecan liposome injections, which are used to treat various diseases such as cancer, vaccines, lung diseases, and fungal infections.
Nanoparticle Drug Delivery
Nanoparticles are a pharmaceutical technique used to improve the solubility of poorly soluble drugs by micronizing the drug substance into nanoparticles, forming a submicron colloidal dispersion system without a carrier. Nanoparticle technology works through several mechanisms: 1) It enhances dissolution rate and solubility. By nanosizing the drug, the surface area is increased, leading to an enhanced dissolution rate for BCS Class II drugs (high permeability, low solubility), significantly improving the drugability of poorly soluble drugs. 2) It offers multiple absorption mechanisms, including passive transport in molecular form, lymphatic absorption, and intercellular or transcellular transport. The advantages of nanoparticle technology are: 1) High safety, as it does not use carriers and contains no significant amounts of surfactants or carrier materials. 2) High bioavailability due to increased surface area through micronization. 3) Enhanced drugability, particularly suitable for Class II drugs in the BCS system. 4) High drug-loading capacity, with no need for additional excipients other than essential stabilizers. Over 20 nanoparticle products have been launched, with administration routes including oral and injection, addressing indications such as pain management, schizophrenia, antifungal, and anti-inflammatory treatments.
3D Printing Drug Delivery
3D printing is a technique that generates three-dimensional solid objects layer by layer from digital designs, with various applications in pharmaceutical formulations. First, immediate-release formulations can be prepared as porous tablets using 3D printing, allowing for rapid drug release, suitable for drugs requiring quick onset. Second, controlled-release formulations can be designed with specific geometric shapes and internal structures to control the rate and pattern of drug release. Additionally, 3D printing can be used to manufacture personalized implants for precise drug delivery. In combination formulations, 3D printing can tailor formulations containing multiple drug components, optimizing drug release characteristics. Topical formulations such as microneedle patches and local delivery systems can also be achieved using 3D printing for precise local delivery. Compared to traditional pharmaceutical technologies, 3D printing offers significant advantages: personalized drug formulations, precise dosage control, and flexible design of complex internal structures and geometric shapes, thereby optimizing drug release behavior.
Microsphere Drug Delivery
Microspheres are small spherical or quasi-spherical particles formed by dissolving or dispersing drugs within polymer materials, with particle sizes ranging from 1 to 250 microns. After injection, the polymer slowly erodes and degrades in the physiological environment, releasing the encapsulated drug gradually as the microspheres degrade. Common polymers used include lipid, PLGA, PLA, PCL, and PGA, with advantages such as long-lasting effects, safety, targeting ability, and specialization. Microsphere technology has been successfully applied in sustained-release formulations for various drugs, including leuprolide, octreotide, and risperidone, used in the treatment of diseases such as cancer, schizophrenia, pain management, and diabetes.
Drug Delivery Platform
BOC Sciences offers comprehensive support services through its advanced drug delivery platform, specializing in the preparation of liposomes, lipid nanoparticles (LNPs), and PEG hydrogels. Our cutting-edge technology enables the production of liposomes for efficient drug encapsulation, enhancing stability and bioavailability, widely used in cancer therapeutics and vaccine development. Additionally, our LNP preparation services focus on nucleic acid drug delivery, such as mRNA vaccines, ensuring precision targeting and efficacy. For sustained release and localized delivery applications, we provide high-performance PEG hydrogel preparation to meet diverse drug delivery needs. At BOC Sciences, we prioritize innovation to empower our clients in advancing drug development and applications.
Liposome Preparation Services | PEG for Drug Delivery |
Lipid Nanoparticles Development | PEG for Hydrogel |
Lipid for Drug Delivery | PEG for Genomics Delivery |
Lipid for Vaccine Delivery | PEG for 3D Bioprinting |
Drug Delivery Routes
The selection of drug delivery routes must take into account the drug properties, therapeutic goals, patient conditions, and disease types. Oral administration, due to its convenience, cost-effectiveness, and high patient acceptance, is the preferred route for most drugs. However, for patients with swallowing difficulties or gastrointestinal diseases, oral administration may not be suitable, making other routes more important. Intravenous injection, due to its rapid onset, is widely used in emergency and critical care treatments. Intramuscular and subcutaneous injections are suited for long-term treatments, such as vaccinations and hormone therapies. Transdermal drug delivery systems slowly release drugs through the skin, commonly used for pain management and the treatment of skin conditions. Inhalation drug delivery quickly delivers drugs via the respiratory tract, especially for treating respiratory diseases such as asthma and chronic obstructive pulmonary disease (COPD). Additionally, specific routes for targeted delivery, such as rectal, nasal, and ocular delivery, provide effective treatments for particular diseases.
Nasal Drug Delivery
Nasal drug delivery involves directly administering drugs into the nasal cavity for therapeutic purposes, accounting for about 2% of all delivery routes. Despite its relatively low proportion, it has significant advantages in local treatments for conditions like rhinitis and nasal congestion. First, nasal delivery avoids the "first-pass effect," where drugs are metabolized by the liver after oral ingestion, reducing their effective concentration in systemic circulation. Nasal delivery directly enters the bloodstream, significantly improving bioavailability. Furthermore, the nasal cavity has minimal enzymatic activity, reducing the risk of drug degradation in the gastrointestinal tract, ensuring drug effectiveness. Additionally, nasal delivery provides rapid onset due to the rich vascularity of the nasal mucosa, making it particularly suitable for emergency treatments. Oxytocin is a typical drug administered nasally, effectively facilitating labor and providing rapid and efficient childbirth support.
Pulmonary Drug Delivery
Pulmonary drug delivery uses devices such as nebulizers, metered-dose inhalers (MDI), or dry powder inhalers (DPI) to deliver drugs in aerosol, mist droplets, or dry powder form to the lungs. This method directly delivers drugs to the site of disease, increasing local drug concentrations and reducing systemic side effects. Pulmonary delivery offers rapid onset as drugs can quickly enter the bloodstream through the alveoli, bypassing the liver's first-pass effect, making it ideal for conditions that require quick symptom control, such as respiratory diseases. Due to the rich capillary network in the lungs and the thin alveolar walls, drugs are efficiently absorbed, improving bioavailability. Moreover, pulmonary delivery reduces side effects associated with injections and oral administration, minimizing drug distribution in other body parts. It is widely used in treating asthma, COPD, and respiratory infections, effectively alleviating symptoms and improving lung function.
Transdermal Drug Delivery
Transdermal drug delivery, also known as percutaneous or skin drug delivery, refers to the process where drugs are absorbed through the skin's stratum corneum and dermis, entering the bloodstream to produce local or systemic therapeutic effects. This method offers advantages such as convenience, simplicity, and prolonged drug effects, making it particularly suitable for patients requiring long-term treatment or those who cannot take oral drugs. Drugs suitable for transdermal delivery typically have both lipophilic and hydrophilic properties, low molecular weight, low melting points, and are non-irritating or non-allergenic to the skin. For example, nitroglycerin, a cardiovascular drug that prevents angina attacks, is delivered via a transdermal patch. This method avoids the first-pass effect and enhances drug bioavailability. The patch is easy to use and provides continuous, stable drug release, effectively controlling angina symptoms.
Controlled Drug Delivery
Traditional drugs often require multiple doses per day to maintain therapeutic drug concentrations, leading to fluctuations in blood levels and potentially reducing efficacy. In contrast, controlled-release systems offer significant advantages by releasing therapeutic doses at a predetermined rate, maintaining drug levels within the ideal therapeutic range, extending treatment duration, and maximizing the drug's effect. Furthermore, controlled-release systems help reduce side effects, decrease dosing frequency, and improve patient adherence to treatment. This innovative technology not only optimizes drug treatment but also significantly enhances the patient's experience and quality of life. Common controlled-release systems include rate-controlled delivery systems, activation-regulated delivery systems, and feedback-controlled delivery systems.
Oral Drug Delivery
Oral drug delivery refers to drugs being taken orally, absorbed through the digestive system into the bloodstream, and exerting their therapeutic effects. It is one of the most commonly used and convenient routes in drug therapy. Oral administration offers benefits such as good patient compliance, high safety, and ease of use, making it widely applicable in the treatment of various diseases. One emerging oral drug delivery method is colonic targeting, designed to deliver drugs directly to the colon for local treatment or to enhance drug bioavailability. This method takes advantage of the unique physiological environment of the colon, such as lower digestive enzyme activity, longer transit time, and higher pH, thus preventing drug degradation and premature release in the stomach and small intestine.
Ocular Drug Delivery
Modern ocular drug delivery systems not only include traditional eye drops and ointments but also incorporate more advanced and complex methods such as ocular implants, eye gels, and eye sprays. These novel delivery systems not only improve drug bioavailability and therapeutic outcomes but also reduce dosing frequency and patient discomfort. The advantage of ocular drug delivery lies in its directness and targeting. Since the drug directly acts on the eye tissue, it can quickly reach the therapeutic site. Additionally, ocular delivery reduces systemic side effects as the drug is primarily absorbed and metabolized locally in the eye, avoiding widespread distribution and potential toxicity in other parts of the body.
Cellular Drug Delivery and Targeting
Cellular drug delivery and targeting technologies utilize cellular or nanoparticle carriers to specifically deliver drugs to target cells or tissues, enhancing therapeutic efficacy and reducing side effects on healthy tissues. This technology typically relies on two methods: one involves receptor-mediated transport systems through the cell membrane, and the other uses nanoparticles or small carriers, such as liposomes or polymer nanoparticles, to carry drugs directly into target cells. The key to cellular drug delivery lies in selecting the appropriate carrier. For example, tumor cells often express specific receptors on their surface, and a drug delivery system can target these receptors to achieve precise targeting. In such cases, the drug is directed to the target cells through interaction with the receptors, effectively increasing drug accumulation and reducing toxicity to normal cells. Furthermore, targeted delivery systems can enhance drug uptake by utilizing specific channels or proteins on the cell membrane, facilitating endocytosis and improving drug accumulation and efficacy inside the cells. With the development of nanotechnology and genetic engineering, cellular drug delivery has become an important tool in precision medicine, particularly in fields like cancer therapy, antiviral treatment, and gene therapy, offering new therapeutic strategies.
Cancer Drug Delivery and Targeting
Cancer drug delivery and targeting technologies aim to deliver drugs precisely to tumor tissues, enhancing therapeutic effectiveness while minimizing toxicity to healthy tissues. Traditional cancer treatments, such as chemotherapy, often result in severe side effects due to the widespread distribution of drugs throughout the body. Targeted drug delivery technologies overcome this problem by optimizing the drug's delivery path. Cancer drug targeting systems commonly rely on nanotechnology, antibodies, ligands, or specific chemical modifications to ensure drugs are accurately targeted to cancer cells. Nanoparticles, especially liposomes, polymer microspheres, and solid lipid nanoparticles, can be designed to recognize specific receptors or antigens on the surface of tumor cells, improving drug targeting and bioavailability. These carriers not only effectively encapsulate drugs but can also optimize drug release behavior by adjusting parameters like particle size, surface charge, and hydrophilicity. Moreover, cancer cells often have a unique microenvironment compared to normal cells, such as an acidic environment and high molecular weight glycosaminoglycans. These features provide new directions for targeted drug design. By exploiting these tumor-specific characteristics, drugs can be released in these specific environments, further enhancing treatment selectivity and efficiency.
Reference
- Gunay, M.S. et al. Drug Delivery Systems for Imaging and Therapy of Parkinson's Disease. Curr Neuropharmacol. 2016, 14(4): 376-91.
Why BOC Sciences?
-
Large Stock
More than 2000+ products in inventory
-
Global Delivery
Warehouses in multiple cities to ensure fast delivery
-
mg to kg
Multi specification for academic research and industrial production
-
24/7 Technical Support
Strict process parameter control to ensure product quality
Technical Support
- Aqueous Two-Phase System (ATPS) Technique
- Capillary Electrophoresis (CE) Technique
- Enzyme-linked immunosorbent assay (ELISA) Technique
- High performance liquid chromatography (HPLC) Technique
- Hydrophobic Interaction Chromatography (HIC) Technique
- PEGylated Protein Purification Techniques
- Radiolabeling Technique
- SDS-PAGE Technique
- Ultrafiltration Technique
Products
- Lipids
- PEG Derivatives by Structure
-
PEG Derivatives by Functional Group
- Acrylate/Acrylamide/Methacrylate PEG
- Aldehyde (Ald/CHO)PEG
- Alkyne PEG
- Amino PEG, PEG amine(-NH2)
- Azide PEG, Azido PEG(-N3)
- Biotin PEG
- Boc/Fmoc protected amine PEG
- Carboxylic Acid(-COOH) PEG
- Cholesterol PEG
- DBCO PEG
- DNP PEG
- DSPE PEG
- Epoxide glycidyl ether PEG
- FITC PEG
- Folate PEG
- Halide (chloride, bromide) PEG
- Hydrazide PEG
- Hydroxyl(-OH) PEG
- Maleimide(-MAL) PEG
- NHS ester PEG
- Nitrophenyl carbonate (NPC) PEG
- Norbornene PEG
- Olefin/Alkene/Vinyl PEG
- Orthopyridyl disulfide (OPSS) PEG
- Phosphate PEG
- Rhodamine PEG
- SCM PEG
- Silane PEG
- SPDP PEG
- Sulfonate (tosyl, mesyl, tresyl) PEG
- tert-Butyl protected carboxylate PEG
- Thiol(-SH) PEG
- Vinylsulfone PEG
- PEG Copolymers
-
PEG Raw Materials
- Small-molecule Polyethylene Glycol
- Polyethylene Glycol 1000
- Polyethylene Glycol 10000
- Polyethylene Glycol 1500
- Polyethylene Glycol 200
- Polyethylene Glycol 2000
- Polyethylene Glycol 20000
- Polyethylene Glycol 400
- Polyethylene Glycol 4000
- Polyethylene Glycol 600
- Polyethylene Glycol 6000
- Polyethylene Glycol 800
- Polyethylene Glycol 8000
Resources
-
Technical Information
- Aqueous Two-Phase System (ATPS) Technique
- Capillary Electrophoresis (CE) Technique
- Enzyme-linked immunosorbent assay (ELISA) Technique
- High performance liquid chromatography (HPLC) Technique
- How to Perform Polyethylene Glycol (PEG) Modification?
- Hydrophobic Interaction Chromatography (HIC) Technique
- Introduction of Polyethylene Glycol (PEG)
- Ion Exchange Chromatography (IEX) Technique
- PEG for Chemical Synthesis
- PEG for Cosmetic Application
- PEG for Drug Delivery
- PEG for Imaging Diagnosis
- PEG for Pharmaceutical Preparation
- PEG for Tissue Engineering
- PEG Purification Techniques of Plasmid DNA
- PEGylated Protein Purification Techniques
- Polyethylene Glycol (PEG) Modifier Selection Guide
- Radiolabeling Technique
- SDS-PAGE Technique
- Size Exclusion Chromatography (SEC) Technique
- Ultrafiltration Technique
-
Industry News
- Applications of PEG-DSPE: Drug Carriers and Drug Delivery
- Applications of Polyethylene Glycol (PEG) as Medical Devices
- Cholesterol: Definition, Structure, Synthesis, Types and Functions
- Classification of Lipid-Based Vaccine Adjuvants
- FDA approved PEGylated Products
- FDA-Approved Antibody-Drug Conjugates up to 2024
- How are Liposomes Different from Micelles?
- How Lipid Nanoparticles (LNPs) Deliver RNA Drugs?
- Hyaluronic Acid & PEGylated Hyaluronic Acid
- Ionizable Lipids for RNA Delivery
- Lipid Classification and Drug Delivery Systems
- Lipid Formulations: Key Absorption-Enhancing Technologies in New Drug Development
- Lipid-Drug Conjugates (LDCs) for Nanoparticle Drug Delivery
- Liposome in Drug Delivery
- Overview of Liposome Preparation Process
- PEG in Pharmaceutical Preparations (I): Solvents, Lubricants, Adhesives and More
- PEG in Pharmaceutical Preparations (II): Stabilizers, Plasticizers and Modification Materials
- PEG Linkers in Antibody Drug Conjugates and PROTACs
- PEG-DSPE Block Copolymers and Their Derivatives
- PEGylated Drugs: Definition, Structure, Classification and Benefits
- PEGylated RGD Peptides: A Promising Tool for Targeted Drug Delivery
- Pharmacokinetics and Bioanalysis of PEGylated Drugs
- Polyethylene Glycol (PEG) Modified Targeting Nanomaterials
- Preparation Method of PEG Hydrogel
- The PROTAC Technology in Drug Development
- Vaccines: Definition, History, Ingredients, Types and Mechanism of Action
- What are Lipid Excipients and Their Applications?
- What are Lipid Nanoparticles and Their Applications?
- What are Lipid-Drug Conjugates (LDCs)?
- What are Lipids?
- What are Monodispersed and Polydispersed PEGs?
- What are PEG Lipids?
- What are Phospholipids?
- What are Sterols? - Definition, Structure, Function, Examples and Uses
- What is Biotinylation and Biotinylated PEG?
- What is Click Chemistry?
- What is Hydrogel?
- What is Methoxy Polyethylene Glycol (mPEG)?
- What is Nanogels and Its Applications?
- What is the Formulation of Lipid Nanoparticles (LNPs)?
Our Feature
BOC Sciences supplies a unique variety of PEG derivatives and functional PEG polymers. Our products offer the most diverse collection of reactivity, ready-to-use functionality, and molecular weight options that you will not find anywhere else.
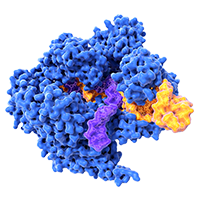
PEGylation of Peptides
and Proteins
Reduce the Immunogenicity of Peptide/Protein Drugs
Learn More
APPLICATIONS
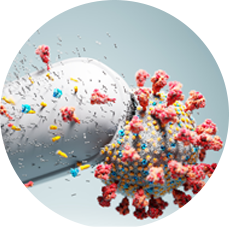
PEG linkers For Drug
Improved Circulation Half-Life
Learn More
Have Customer Reviewed On Us?
Chat With Us